Breast volumetric analysis for aesthetic planning in breast reconstruction: a literature review of techniques
Introduction
Accurate assessment of breast volume is an essential component of preoperative planning in both reconstructive and aesthetic breast operations for achieving breast symmetrization and satisfactory outcome (1-5). Breast shape is dynamic and highly dependent on patient position, and is also highly variable between patients; therefore any objective method of volumetric analysis requires a high degree of versatility. However, an accurate and reliable method of objective breast volumetric analysis, that is also clinically useful, has been elusive (6). Currently, most surgeons rely on visual estimation and two-dimensional (2D) photographs to evaluate breast volume that is irreproducible and is subject to individual clinical experiences. An ideal breast volume assessment technique should be accurate, reliable, simple, and practical.
Since the earliest report of breast volume measurement by Bouman (7), various techniques have been described of varying accuracy and reliability: water displacement technique using Archimedean principle, negative molding using thermoplastic casts, direct anthropomorphic measurements, indirect anthropomorphic measurements using 2D imaging and standard (or 3D) imaging, and 3D surface scanning technology (2,8-23). Countless number of comparative studies (6,13,18,24-37) and reviews of individual techniques (38,39) have been described. However, a unifying, updated review of all techniques comparing their accuracy, reliability and practicality has been lacking (6,24,40).
In this review, we summarize all the currently available techniques calculating breast volume and compare their accuracy, reproducibility, and practicality.
Methods
We reviewed the published English literature from 1950 to 2015 from well-established databases, such as PubMed, Medline, Web of Science, and EMBASE, using various combination of search terms, such as “breast volumetric analysis”, “breast reconstruction”, “breast asymmetry”, “aesthetic breast surgery”, “anthropomorphic”, “mammography”, “Archimedean”, “thermoplastic”, “computed tomographic angiograph”, “magnetic resonance imaging (MRI)”, “3D surface scanning”, and “3D printing”.
Results/discussion
Various techniques for breast volumetric analysis have been reported in the literature, such as Archimedean principle, thermoplastic casting, anthropomorphic measurement, 2D imaging, 3D imaging, and 3D surface imaging (Tables 1,2).
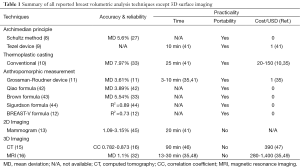
Full table
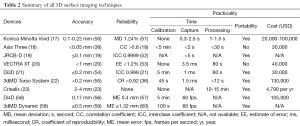
Full table
Archimedean principle
First reported by Bouman in 1970 (7), water displacement technique works on the Archimedean principle where the volume of water displaced equals the volume of breast submerged in water (Table 1) (8,61). Discouragingly, the earlier method described by Schultz et al. (8) are wet and messy, require complete patient cooperation, and cannot be performed intraoperatively. To this effect, investigators have devised various forms of calibrated measurement cylinders containing liquid with a flexible diaphragm at the base (9,44,62-65). In contrast to commercial devices (63,65), Tezel and Numanoglu describe a free, easy-to-assemble device that can be assembled from equipments that can be readily found on a sterile operating table (9). In comparison to the gold standard mastectomy specimens, water displacement technique shows only ~2% difference (36). However, against mammography-derived volume estimation, it correlates less favorably (regression coefficient =0.608) (6). Major disadvantages of the Archimedean techniques are that the breast tissue lateral to pectoral fold is often missed leading to underestimation in hypertrophic breasts.
Thermoplastic casting
Thermoplastic cast method uses a fast-setting plaster or a thermoplastic sheet that is applied on to the thoracic wall and creates a negative mold of the breasts, from which volume can be measured with either sand or water (Table 1) (6,10,37). Despite its advantages, such as it can be performed with patient sitting upright and the breast shape can be visualized additionally, it has numerous failings that prevent it from being used routinely. It is a subjective technique and during the process of manually pressing the thermoplastic material to the thorax, the breast becomes compressed and its boundaries become arbitrary, making it difficult to determine the breast footprint reliably. Moreover, if the material is relatively inflexible, it cannot mold perfectly around the breast form. It incurs high material cost ($150 per breast) and has a relatively poor accuracy (coefficient variable =6%) (10).
Anthropomorphic measurement
Anthropomorphic measurement is one of the earliest methods of calculating breast volume that is still used by physicians (Table 1). A volume is derived from a mathematical formula using predefined end-to-end measurements that are obtained either directly from the patient or indirectly via imaging modalities. In order to define a set of “ideal” measurement values for comparison, Penn has derived parameters from “aesthetically perfect” breasts, which, however, did not correlate well clinically (66). Smith et al. have studied 55 consecutive women to determine a set of “average” measurements, but they utilized soft tissue parameters that are difficult to reproduce (67). Later, Westreich et al. has described a standardized protocol for assessing female breasts using a measuring tape and a Grossman-Roudner device, achieving a mean measurement deviation of only 3.61% (11). Grossman-Roudner device is an adjustable cone made out of flat envelope that changes to the size of the breast and volume can be read off a scale (68). Similarly, Palin et al. have used a set of graduated discs to calculate volume (40). However, the Palin discs only work for volumes up to 450 mL and overestimates in firm breasts. More lately, Sigurdson and Kirkland have introduced an equation to predict volume based on two anthropomorphic variables that significantly correlated with the volumes measured by the water displacement method, demonstrating high accuracy (R2 =0.89) in 101 normal female volunteers (44).
In contrast to the conventional formulae that have been standardized against water displacement techniques, Qiao et al. report a modified method where a breast is equated to a half-ellipse and the parameters of the mathematical formula of half-ellipse are applied to estimate volume (42). The authors have applied the formula to 125 Chinese women and reported a mean measurement deviation of 3.89% (42). This is reproduced by Bulstrode et al., demonstrating its high correlation with mammogram-derived volume measurements (R2 =0.830) (6). However, the Qiao formula is more appropriate for smaller conical-shaped breasts and its accuracy is significantly reduced in hypertrophic cylindrical breasts (44). In 2013, Longo et al. have introduced the BREAST-V formula that has been derived by correlating anthropomorphic measurements against mastectomy specimen weight in 88 women undergoing modified radical mastectomy (12). It is a relatively simple and objective method only requiring three parameters. The authors demonstrate that the formula is significantly superior to the Sigurdson formula from their own experience (R2 =0.73 vs. 0.55). However, it remains to be validated in a larger trial.
Major advantages of direct anthropomorphic measurements are that they are relatively easy to perform, cheap, require minimal apparatus, and can be measured with patient standing up. However, since most mathematical formulae used rely on imposing certain geometrical shapes on to breasts, they ignore the wide variability and individuality in breast shapes. Furthermore, most anatomical landmarks chosen as parameters can be subjective and arbitrary. Where anatomical landmarks and submammary regions are not well-defined, accuracy can be significantly compromised (17).
Imaging
2D imaging
Various 2D imaging modalities have been utilized, from which anthropomorphic variables can be derived and estimate breast volume, mainly mammography (13), 2D photography (69,70), and 2D ultrasound (14) (Table 1). A major advantage of utilizing imaging is that measurements and calculations can be conducted away from the patient, which can lead to objective assessment and standardization. Furthermore, imagings like mammograms are already being performed routinely for breast cancer screening (71). Applying parameters of the mathematical formula of cone on mammograms, volumes can be estimated to the accuracy of 1.09-3.15% (45). Unsurprisingly, due to radiation exposure, it is the least acceptable volumetric analysis tool by the patients (6) and is not indicated for utility in benign breast conditions, such as Poland syndrome. Brown et al. have utilized 2D photographs, to which they have applied the Qiao formula and calculated volume (43). However, 2D photographs fail to adequately demonstrate depth and shape, which significantly limits its accuracy (72). Similarly, an ultrasound probe has been used to scan a breast on either sagittal or transverse slices, from which an “area” of the breast is calculated and summed to yield a volume (14). However, it has a mean measurement deviation of 8% (14).
3D imaging
In contrast to other breast volumetric analysis techniques, the conventional 3D imaging modalities, such as computed tomography (CT) and MRI, enable visualization of internal structures leading to potentially more accurate estimation of the breast parenchymal volume (Table 1) (6,10,15,16,32,33,35,40,49,73-76). Furthermore, CT and MRI data are usually readily available since they are routinely performed as preoperative planning tool in autologous breast reconstructions and for diagnosis of breast cancers, respectively. Using a computer software either manually by an operator or automatically by an underlying mathematical algorithm, a region of interest encompassing the breast tissue can be segmented on individual axial slices. Given that all slices have equal thickness, the sum of all segmented volumes indicate the total parenchymal volume. Kim et al. have compared the modalities and demonstrated that MRI is more accurate than CT in determining the volume of the resected breast tissue (0.928 vs. 0.782; P=0.001) and the final autologous flap (0.959 vs. 0.873; P=0.001) (16). Moreover, MRI has a reported mean measurement deviation of only 4.3% (75). This is most likely related to the superior soft tissue resolution in MRI. Furthermore, CT scans involve radiation exposure, making them an unattractive option. In contrast, MRI does not involve radiation or require the use of intravenous contrast, and it can be performed in prone position facilitating accurate definition of the breast boundaries and its shape. Major disadvantages of MRI are its cost ($1,400 per scan) (35), it is time-consuming, and is contraindicated in patients with claustrophobia. Moreover, segmentation on imaging software is still mostly conducted manually, which is labor-intensive, and evidences supporting commercially available automatic segmentation tools are scarce (76,77).
3D surface imaging
3D surface imaging is the latest and the most extensively studied technique of breast volumetric analysis (Table 2). It describes a technology where the light reflected off a surface is captured to build a virtual 3D model, from which both quantitative and qualitative analysis of volume and shape can be deduced. 3D surface scanning has been utilized in the automotive and aerospace industry for decades where accuracy is paramount. One of the first uses of this technology in medical application has been evaluation of facial asymmetry in orthognathic conditions (78-84). In breast evaluation, Galdino et al. have first used 3D surface scanners to quantitate parameters, such as volume and shape, to assess symmetry (2). Interestingly, a follow-up study by the same authors fail to show any clinical improvement in achieving volumetric symmetry after autologous breast reconstructions despite using 3D scanners during preoperative planning (85). However, the authors note that the approach was not standardized and the anatomical landmarks were inconsistent (85).
Early 3D surface imaging techniques utilized by clinicians have been cumbersome and unreliable, such as image-subtraction technique (86), liquid-crystal scanning (87), light-luminance scanning (88), basic laser scanning (89-91), stereolithography (92), video system (93-96), and moiré topography (31,97,98). Recent advances in optical imaging systems and software developments facilitated by growing databases of anatomical profiles, 3D surface scanning has become more accurate and reliable, and the patient protocols have become simplified (38,99,100). For clinical application, laser imaging, structured light and stereophotogrammetry 3D scanning techniques have been most commonly studied (Table 2).
Laser imaging technique
The latest laser imaging technology projects a certain pattern of laser (i.e., spot and stripe) to a surface, which is captured by a calibrated camera placed at a known triangulation distance from the laser source (50). Triangulation is a mathematical calculation used to derive at 3D coordinate (i.e., x, y, and z) of a point on the object surface where the laser has hit (100). In contrast to the optical techniques, laser scanning technology can produce a more regular grid of points (50). However, it is less sensitive to edge effect, occlusion, and sharp transition in depth (50). One of the limitations of laser scanners is their inability to differentiate the posterior surface of the breasts from the chest wall and image 360 degrees. To this effect, proprietary software have been standardized to recognize breast boundaries according to the “mammometric” parameters defined by Tepper et al. (101), and subsequently accurately estimate the posterior surface (51). In addition, rotating subjects on a turntable to image 360 degrees, as seen with inanimate objects in industrial practice, is not feasible in clinical application without creating significant motion artifacts. To this effect, Tepper et al. have demonstrated that standing patients with arms by their side in anatomical position and introducing fluorescent illumination that reduces shadowing effect improve visualization (102).
Currently, numerous commercial 3D laser scanners are available, such as Cyberware Whole Body Color 3D Scanner (Cyberware, Monterey, CA, USA) (27,28). However, Konica Minolta 3D scanners (Konica Minolta Inc., Tokyo, Japan) are the most extensively investigated one in the literature (1,17,32,33,51,101-108).
(I) Konica Minolta 3D scanner
Konica Minolta Vivid 910 3D scanners contain a linear laser scanner with a reported accuracy of 0.1-0.22 mm (50) and high reproducibility (51,109) (Table 2). Kovacs et al. report that the technique most reliably measures breast volumes between 300 and 1,600 mL (51) and it has a high correlation with MRI (33). In addition, its reliability can be improved by connecting two or more scanners together reducing motion artifacts, however, multiplying costs (17). For ptotic and hypertrophic breasts, obtaining multiple views and manually merging them together on the accompanying free computer software facilitates accurate volumetric analysis (17). Major advantages of the Konica Minolta scanners are that they are simple to operate, require no calibration, have a fast capture and processing speed, and are portable (11 kg). However, its price (USD 20,000-100,000) is a significant drawback, especially where more than one device are necessary. To date, Konica Minolta scanners have been used to facilitate preoperative planning and postoperative monitoring in implant breast reconstruction (1), reduction mammoplasty (107), and breast augmentation (108).
Structured light technique
When an organized pattern of light (i.e., stripe, grid, and dots) is projected on to an object, it becomes distorted in a predictable manner. Structured light technology utilizes a camera system calibrated to the predicted distortion of the light patterns to capture and generate a 3D surface data (99,110). Placing cameras at two separate viewpoints further eliminates pattern interference (99). Numerous non-commercial devices, such as MTV camera (Mintron, New Taipei City, Taiwan, China) (111), Daly Shape Measurement System (112), Malata Bodymap System (113), and Precision Light Imaging System (114-116), and commercial units, such as CAM3D (3D-Shape, Erlangen, Germany) (29), C3D (C3D, Beirut, Lebanon), Voxelan (Hamano Engineering, Kanagawa, Japan) (34), and Rainbow 3D Camera (Genex Technologies Inc., Kensington, MD, USA) (2), have been described. However, the most frequently studied structured light device is Axis Three scanner (Axis Three, Miami, FL, USA).
(I) Axis Three scanner
Axis Three Torso System extrapolates 3D data using color-coded triangulation (CCTTM) technique conceived from collaboration between Axis Three and Siemens (Siemens Technology Accelerator, Munich, Germany) (Table 2). CCTTM consists of sequential firing of images from three separate cameras and has a reported accuracy of 0.05 mm. The system requires calibration (less than 5 min) every time it is physically relocated and has a relatively slow capture (2 sec) and processing speed (30 sec). Interestingly, Axis Three has developed a physics-driven tissue behavior simulation (TBS) software program using its database of real patients. Furthermore, a physician can individualize the tissue elasticity to the patient in real-time while navigating the program in a consulting room. An initial report suggests that the software quality may be compromised due to the relatively slow image acquisition speed of the hardware (100). Studying 22 patients undergoing breast augmentation, Mailey et al. demonstrate that mean difference between the simulation and the actual outcome is 12% (range, 0.4-30%), but the system still has high reproducibility (correlation coefficient of >0.8). To date, more studies are required to validate this technology.
Stereophotogrammetry
Stereophotogrammetry is an imaging method similar to the human eye physiology where a stereo pair of cameras shoots a single object from multiple angles and their points of intersections are recorded (100,117,118). 3D surface data is rendered using triangulation technique where 3D coordinates are calculated for each 2D surface point. Depending on the presence of an additional light source being projected on to the object, stereophotogrammetry technology can be classified into active, passive, and hybrid (38).
(I) Active stereophotogrammetry
In active stereophotogrammetry, the surface information is gathered from two sources: random, unstructured light being projected on to the object and natural reflections from the innate patterns on the object’s surface. The inclusion of projected light makes it easier for the software to compute triangulation and resists interference from ambient lighting. The most frequently studied commercial device using active stereophotogrammetry is JRCB-D 3D scanner (Jirui, Beijing, China) (19,52,119,120).
(i) JRCB-D scanner
JRCB-D system consists of two paired cameras that capture light illuminated by a grating projector (Table 2) (19). The standard protocol requires patients to stand leaning against a flat wall and their hands on the anterior superior iliac spine, also known as akimbo. The wall is also captured and analyzed to help generate the x-y Cartesian plane. Using ideal models from virtual breast augmentation, Liu et al. demonstrate that a JRCB-D scanner calculate volume to 1 mL accuracy and its analysis is reliable (interclass coefficient of 0.9999) (52). Interestingly, the same group reports that maintaining patients in the same respiratory state during image capture is also critical (119). To date, the technology has been used for preoperative assessment (19) and postoperative monitoring in breast augmentation (120).
(I) Passive stereophotogrammetry
A 3D imaging system using passive stereophotogrammetry lacks the projected light source and relies alone on the natural reflections from the innate patterns on an object’s surface. As a result, a requirement for high-quality cameras is crucial to achieving adequate detail. Similarly, choosing an object to visualize that has sufficient surface textures (e.g., pores, freckles, scars, and rhytids) is essential. Furthermore, ambient lighting must be carefully controlled and identifying 3D coordinates for triangulation by the software is comparably more difficult. The most commonly used commercial passive stereophotogrammetry devices are VECTRA scanners (Canfield Scientific, Fairfield, NJ, USA) (20,53,121) and Di3D scanners (Dimensional Imaging, Glasgow, Scotland) (21,30,54,122,123).
(i) VECTRA scanner
VECTRA XT scanners are modular 3D imaging systems equipped with three stereo pods containing two color single-lens reflex (SLR) cameras each. These are capable of capturing high-resolution photos at a fast speed (3.5 milliseconds) (Table 2). VECTRA systems are accompanied by two types of software. Mirror® medical imaging (Canfield Scientific, Fairfield, NJ, USA) is a well-respected patented program that simulates surgical procedures on 2D photographs. Canfield Sculptor® is the latest suite of programs that enables tissue simulation on 3D reconstructed surface images. Namely, the Breast Sculptor® software is capable of performing automatic measurements and simulates outcomes of virtual breast augmentation procedures. Using faces of normal adult subjects, de Menezes et al. report that VECTRA scanners are accurate with low mean measurement error (<1 mm) and are reliable, as demonstrated by negligible intra- and inter-observer variability (P>0.05) (20). Similarly, Rosati et al. show that using VECTRA scanners to compare measurements on virtual dental models to in vivo, the estimate of error is less than 1.2% and there are also no significant differences between repeated measurements (53). In a single-blinded study, Roostaeian et al. have compared the software simulation to the actual patient measurements after primary breast augmentation procedure and demonstrate a mean accuracy of 90.8% (26). Moreover, VECTRA scanners have been used to illustrate the “bottoming out” phenomenon after breast reduction (121). Major limitations of VECTRA systems are its high cost, relatively slow image processing speed, and lack of portability (Table 2).
(ii) Di3D scanner
Di3D scanners utilize four high-quality digital SLR (DSLR) cameras, Canon EOS 550D (Canon Inc., Tokyo, Japan), to generate ultra-high quality 3D images (Table 2). The system has been used extensively in the entertainment industry by video game producers. Unique features of Di3D include the optional capability in the proprietary software that combines CT scan data with the 3D surface images, and the revolutionary 4D imaging technology by Di4D (Dimensional Imaging, Glasgow, Scotland) that captures dynamically changing anatomical structures. Earlier studies using Di3D scanners on facial models have shown that its accuracy is clinical acceptable and reliable (repeatability error of 0.0016 mm) (54,122). In a comparative study using cadaver heads, Fourie et al. demonstrate that Di3D system is as accurate and reliable as Konica Minolta laser 3D scanners and CT scans (123). Catherwood et al. are the first to report its use on breasts demonstrating that it is reliable (interclass coefficient of 0.999) and the linear measurements derived from the 3D surface data are accurate (<0.5 mm) (21). To date, no clinical application of this technology in breast surgery has been reported.
(I) Hybrid stereophotogrammetry
Hybrid stereophotogrammetry combines active and passive stereophotogrammetry to achieve higher accuracy and image quality. Currently, the most well-studied hybrid scanner is 3dMD scanner (3dMD, Atlanta, GA, USA) (22,30,36,55,124-129).
(i) 3dMD scanner
3dMDtorso system consists of four modular units of 12 synchronized machine vision quality cameras that are of engineering and industrial standards and are superior to the regular SLR cameras (Table 2). The accompanying software has novel functions, such as the ability to track patient history and simulate soft tissue behaviors using biomechanical mass-spring method (125). Furthermore, there are optional additional software, such as 3dMDvultus that allows 3D image fusion with CT data and 3dMDdynamic that facilitates 4D imaging. 3dMDtorso system has a reported accuracy of 0.2 mm (range, 0.1-0.5 mm) (55,127) and high reliability as depicted by low intra- and inter-observer errors (1.2 and 1.0 mm, respectively) (126). Moreover, its high capture speed (1.5 milliseconds) makes it resistant to motion artifacts. In breast volumetric analysis, Losken et al. have applied 3dMD system in the preoperative work-up for skin-sparing mastectomy and demonstrate that the mean difference between the calculated and the actual volume is only ~2% and the coefficient of reproducibility is less than 0.92 (36). In addition, Henseler et al. report that the 3D imaging system is superior to the traditional water displacement technique in accuracy (P≤0.017) and reproducibility (36 vs. 62.6 units/cc) (30). To date, clinicians have utilized 3dMD scanners to demonstrate baseline breast asymmetry in 87 normal women (124) and study the compressive effect of implants on breast volume after augmentation (22). Significantly, in the latter study, the authors report no difference between the imaging-derived expected breast volume and the actual augmented volume (P=0.3483) (22). Despite its fast processing speed and mobility, a major limitation of the imaging system is related to its high cost.
Novel 3D imaging technologies
Crisalix
Crisalix (Crisalix, Lausanne, Switzerland) is the first, web-based 3D imaging technology that generates 3D surface data from three 2D photographs (front, left, and right) taken by patients on their own consumer cameras (Table 2). In addition, a user needs to upload the physical distance from which the photo was taken and a set of anatomical landmarks. Calculation and simulation is performed via a cloud computing storage database based in Switzerland. The program contains two simulation engines, 3D FACE Simulator (130) and 3D MAMMO Simulator (23). The latter enables a patient to examine and choose their preferred choice of breast implant size and brands prior to attending an aesthetic plastic surgeon’s consultation. In 11 cases of women planning for breast augmentation, de Heras Ciechomski et al. compared Crisalix program and a consumer handheld 3D laser scanner, EScan 3D (3D Digital Corp, New Town, CT, USA) (23). The program performs comparably to the laser scanner and reports a mean error of 2-4 mm (23). Despite its ease of access and a reasonable price, more robust evidence is required to validate this novel technology.
4D imaging
In contrast to static 3D imaging techniques, 4D imaging adds temporal resolution facilitating dynamic analysis of 3D structures, such as breasts (Table 2). In the literature, numerous 4D imaging systems have been used to study mainly facial animation and asymmetry (38,59,131). The most commonly investigated commercial 4D system has been Di4D (Dimensional Imaging, Glasgow, Scotland) (56,57,132) and 3dMDdynamic (3dMD, Atlanta, GA, USA) (58,60). Di4D scanners consist of two pods with two greyscales and a color digital video camera each. Main advantages of the system are that it requires only the standard video lighting and has an inbuilt optical flow tracking capability with automatized digitization of facial landmarks to track every pixel in every image being shot. However, it remains expensive and is yet to be validated in breast surgery. The latest 3dMDdynamic body system consists of 9-22 modular units of 27-66 machine vision quality cameras and an LED-based lighting system that minimizes interference and yields smooth sequential playback. In addition, the accompanying 3dMDtempus surface tracking software helps analyze skin dynamics reliably from different positions. To date, 3dMDdynamic system has been studied in facial anatomy showing high accuracy and reliability (60). However, it has yet to be applied in breast volumetric assessment.
3D printing
3D printing, also known as additive manufacturing or rapid prototyping, is the latest novel technology to aid clinicians, in combination with 3D imaging, for assessing breast volume (39,76,77,133-135). 3D printing describes a process where a computer-aided design (CAD) is fabricated into an end product in a layer-by-layer fashion (77,133,136-140). The resultant haptic biomodel provides a 360-degree visualization and tactile feedback, enabling a superior visuospatial appreciation of the anatomy (141). In medical application, CAD files can be derived from any conventional imaging source, such as CT and MRI, or 3D surface imaging. Chae et al. have reported a case where routine preoperative CT scan is used to calculate the breast volume differential (Figure 1) and a model of the anterior chest wall is 3D printed to enhance visualization of the asymmetry (Figure 2) (76). As 3D printing becomes more affordable and intuitive to use by physicians, combining 3D printing and 3D imaging may revolutionize preoperative planning in breast reconstruction and the manufacturing of customized breast implants (39,77,134,142).
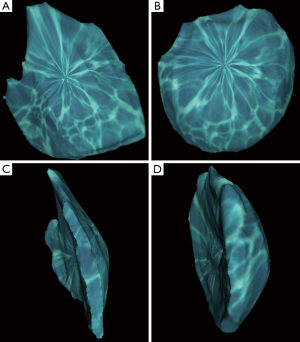
Recently, the concept of 4D printing has been described, in which the fourth dimension, time, is incorporated into the conventional 3D printing by utilizing 4D CT scan data (143). The technique of 4D CT has been initially developed to minimize motion artifacts and enhance the image quality of lungs for accurate assess of lung tumor volume and planning precise delivery of radiotherapy (144). Moreover, using the previously described “single volume acquisition” scanning method, overexposure to radiation is prevented and the overall dose is reduced to 1.78 mSv, equivalent of three plain abdominal X-rays (145). In addition to respiratory medicine, 4D CT has been used in breast cancers, renal tumors, and pancreatic cancers (146-148). In plastic surgery, clinicians have used 4D CT mainly to study perforator vascular dynamics (149-151). In 2015, Chae et al. have used 4D CT for 4D printing the bones of the thumb ray to depict the translation of metacarpal bones during various thumb movements and validated their technique (143). To date, 4D printing has not been applied in breast volumetric analysis. However, studies are currently underway to investigate dynamic changes in breast volume by 3D printing breasts during movement of the arm and torso.
Conclusions
Accurate volume measurement is an integral part of preoperative planning in both reconstructive and aesthetic breast procedures. Before the introduction of 3D scanning, most previous methods have been unreliable, difficult to execute, and had limited practicability. 3D surface imaging has revolutionized the field with its ease of use, reliability, fast speed, and improving portability. However, it is not yet being used widely due to its high cost and lack of published high level of evidence. Recently, interesting advancements and adjuncts to 3D scanning have been introduced, mainly the first web-based 3D surface imaging program, 4D imaging, and 3D printing.
Acknowledgements
None.
Footnote
Conflicts of Interest: The authors have no conflicts of interest to declare.
References
- Tepper OM, Karp NS, Small K, et al. Three-dimensional imaging provides valuable clinical data to aid in unilateral tissue expander-implant breast reconstruction. Breast J 2008;14:543-50. [PubMed]
- Galdino GM, Nahabedian M, Chiaramonte M, et al. Clinical applications of three-dimensional photography in breast surgery. Plast Reconstr Surg 2002;110:58-70. [PubMed]
- Hudson DA. Factors determining shape and symmetry in immediate breast reconstruction. Ann Plast Surg 2004;52:15-21. [PubMed]
- Kovacs L, Zimmermann A, Papadopulos NA, et al. Re: factors determining shape and symmetry in immediate breast reconstruction. Ann Plast Surg 2004;53:192-4. [PubMed]
- Lee HY, Hong K, Kim EA. Measurement protocol of women’s nude breasts using a 3D scanning technique. Appl Ergon 2004;35:353-9. [PubMed]
- Bulstrode N, Bellamy E, Shrotria S. Breast volume assessment: comparing five different techniques. Breast 2001;10:117-23. [PubMed]
- Bouman FG. Volumetric measurement of the human breast and breast tissue before and during mammaplasty. Br J Plast Surg 1970;23:263-4. [PubMed]
- Schultz RC, Dolezal RF, Nolan J. Further applications of Archimedes’ principle in the correction of asymmetrical breasts. Ann Plast Surg 1986;16:98-101. [PubMed]
- Tezel E, Numanoğlu A. Practical do-it-yourself device for accurate volume measurement of breast. Plast Reconstr Surg 2000;105:1019-23. [PubMed]
- Edsander-Nord A, Wickman M, Jurell G. Measurement of breast volume with thermoplastic casts. Scand J Plast Reconstr Surg Hand Surg 1996;30:129-32. [PubMed]
- Westreich M. Anthropomorphic breast measurement: protocol and results in 50 women with aesthetically perfect breasts and clinical application. Plast Reconstr Surg 1997;100:468-79. [PubMed]
- Longo B, Farcomeni A, Ferri G, et al. The BREAST-V: a unifying predictive formula for volume assessment in small, medium, and large breasts. Plast Reconstr Surg 2013;132:1e-7e. [PubMed]
- Katariya RN, Forrest AP, Gravelle IH. Breast volumes in cancer of the breast. Br J Cancer 1974;29:270-3. [PubMed]
- Malini S, Smith EO, Goldzieher JW. Measurement of breast volume by ultrasound during normal menstrual cycles and with oral contraceptive use. Obstet Gynecol 1985;66:538-41. [PubMed]
- Sotsuka Y, Fujikawa M, Izumi K. Volume of deep inferior epigastric perforator flap quantified preoperatively by using 64-multidetector-row computed tomography. J Plast Reconstr Aesthet Surg 2012;65:1601-3. [PubMed]
- Kim H, Mun GH, Wiraatmadja ES, et al. Preoperative magnetic resonance imaging-based breast volumetry for immediate breast reconstruction. Aesthetic Plast Surg 2015;39:369-76. [PubMed]
- Kovacs L, Yassouridis A, Zimmermann A, et al. Optimization of 3-dimensional imaging of the breast region with 3-dimensional laser scanners. Ann Plast Surg 2006;56:229-36. [PubMed]
- Mailey B, Freel A, Wong R, et al. Clinical accuracy and reproducibility of Portrait 3D Surgical Simulation Platform in breast augmentation. Aesthet Surg J 2013;33:84-92. [PubMed]
- Liu C, Luan J, Mu L, et al. The role of three-dimensional scanning technique in evaluation of breast asymmetry in breast augmentation: a 100-case study. Plast Reconstr Surg 2010;126:2125-32. [PubMed]
- de Menezes M, Rosati R, Ferrario VF, et al. Accuracy and reproducibility of a 3-dimensional stereophotogrammetric imaging system. J Oral Maxillofac Surg 2010;68:2129-35. [PubMed]
- Catherwood T, McCaughan E, Greer E, et al. Validation of a passive stereophotogrammetry system for imaging of the breast: a geometric analysis. Med Eng Phys 2011;33:900-5. [PubMed]
- Hill SM, Huettner F, Murray J, et al. Contribution of breast density to the volume of the augmented breast: A preliminary study. Can J Plast Surg 2011;19:93-6. [PubMed]
- de Heras Ciechomski P, Constantinescu M, Garcia J, et al. Development and implementation of a web-enabled 3D consultation tool for breast augmentation surgery based on 3D-image reconstruction of 2D pictures. J Med Internet Res 2012;14:e21. [PubMed]
- Xi W, Perdanasari AT, Ong Y, et al. Objective breast volume, shape and surface area assessment: a systematic review of breast measurement methods. Aesthetic Plast Surg 2014;38:1116-30. [PubMed]
- Yoo A, Minn KW, Jin US. Magnetic resonance imaging-based volumetric analysis and its relationship to actual breast weight. Arch Plast Surg 2013;40:203-8. [PubMed]
- Roostaeian J, Adams WP Jr. Three-Dimensional Imaging for Breast Augmentation: Is This Technology Providing Accurate Simulations? Aesthet Surg J 2014;34:857-75. [PubMed]
- Yip JM, Mouratova N, Jeffery RM, et al. Accurate assessment of breast volume: a study comparing the volumetric gold standard (direct water displacement measurement of mastectomy specimen) with a 3D laser scanning technique. Ann Plast Surg 2012;68:135-41. [PubMed]
- Veitch D, Burford K, Dench P, et al. Measurement of breast volume using body scan technology(computer-aided anthropometry). Work 2012;41 Suppl 1:4038-45. [PubMed]
- Koch MC, Adamietz B, Jud SM, et al. Breast volumetry using a three-dimensional surface assessment technique. Aesthetic Plast Surg 2011;35:847-55. [PubMed]
- Henseler H, Khambay BS, Bowman A, et al. Investigation into accuracy and reproducibility of a 3D breast imaging system using multiple stereo cameras. J Plast Reconstr Aesthet Surg 2011;64:577-82. [PubMed]
- Thomson JG, Liu YJ, Restifo RJ, et al. Surface area measurement of the female breast: phase I. Validation of a novel optical technique. Plast Reconstr Surg 2009;123:1588-96. [PubMed]
- Eder M, Schneider A, Feussner H, et al. Breast volume assessment based on 3D surface geometry: verification of the method using MR imaging. Biomed Tech (Berl) 2008;53:112-21. [PubMed]
- Kovacs L, Eder M, Hollweck R, et al. Comparison between breast volume measurement using 3D surface imaging and classical techniques. Breast 2007;16:137-45. [PubMed]
- Isogai N, Sai K, Kamiishi H, et al. Quantitative analysis of the reconstructed breast using a 3-dimensional laser light scanner. Ann Plast Surg 2006;56:237-42. [PubMed]
- Caruso MK, Guillot TS, Nguyen T, et al. The cost effectiveness of three different measures of breast volume. Aesthetic Plast Surg 2006;30:16-20. [PubMed]
- Losken A, Seify H, Denson DD, et al. Validating three-dimensional imaging of the breast. Ann Plast Surg 2005;54:471-6; discussion 477-8. [PubMed]
- Campaigne BN, Katch VL, Freedson P, et al. Measurement of breast volume in females: description of a reliable method. Ann Hum Biol 1979;6:363-7. [PubMed]
- Tzou CH, Artner NM, Pona I, et al. Comparison of three-dimensional surface-imaging systems. J Plast Reconstr Aesthet Surg 2014;67:489-97. [PubMed]
- Chang JB, Small KH, Choi M, et al. Three-dimensional surface imaging in plastic surgery: foundation, practical applications, and beyond. Plast Reconstr Surg 2015;135:1295-304. [PubMed]
- Palin WE Jr, von Fraunhofer JA, Smith DJ Jr. Measurement of breast volume: comparison of techniques. Plast Reconstr Surg 1986;77:253-5. [PubMed]
- Kayar R, Civelek S, Cobanoglu M, et al. Five methods of breast volume measurement: a comparative study of measurements of specimen volume in 30 mastectomy cases. Breast Cancer (Auckl) 2011;5:43-52. [PubMed]
- Qiao Q, Zhou G, Ling Y. Breast volume measurement in young Chinese women and clinical applications. Aesthetic Plast Surg 1997;21:362-8. [PubMed]
- Brown RW, Cheng YC, Kurtay M. A formula for surgical modifications of the breast. Plast Reconstr Surg 2000;106:1342-5. [PubMed]
- Sigurdson LJ, Kirkland SA. Breast volume determination in breast hypertrophy: an accurate method using two anthropomorphic measurements. Plast Reconstr Surg 2006;118:313-20. [PubMed]
- Kalbhen CL, McGill JJ, Fendley PM, et al. Mammographic determination of breast volume: comparing different methods. AJR Am J Roentgenol 1999;173:1643-9. [PubMed]
- Smit JM, Dimopoulou A, Liss AG, et al. Preoperative CT angiography reduces surgery time in perforator flap reconstruction. J Plast Reconstr Aesthet Surg 2009;62:1112-7. [PubMed]
- Rozen WM, Ashton MW, Whitaker IS, et al. The financial implications of computed tomographic angiography in DIEP flap surgery: a cost analysis. Microsurgery 2009;29:168-9. [PubMed]
- Pozzobon AV, Sabino Neto M, Veiga DF, et al. Magnetic resonance images and linear measurements in the surgical treatment of breast asymmetry. Aesthetic Plast Surg 2009;33:196-203. [PubMed]
- Herold C, Reichelt A, Stieglitz LH, et al. MRI-based breast volumetry-evaluation of three different software solutions. J Digit Imaging 2010;23:603-10. [PubMed]
- Hill DL, Berg DC, Raso VJ, et al. Evaluation of a laser scanner for surface topography. Stud Health Technol Inform 2002;88:90-4. [PubMed]
- Kovacs L, Eder M, Hollweck R, et al. New aspects of breast volume measurement using 3-dimensional surface imaging. Ann Plast Surg 2006;57:602-10. [PubMed]
- Liu C, Luan J, Ji K, et al. Measuring volumetric change after augmentation mammaplasty using a three-dimensional scanning technique: an innovative method. Aesthetic Plast Surg 2012;36:1134-9. [PubMed]
- Rosati R, De Menezes M, Rossetti A, et al. Digital dental cast placement in 3-dimensional, full-face reconstruction: a technical evaluation. Am J Orthod Dentofacial Orthop 2010;138:84-8. [PubMed]
- Khambay B, Nairn N, Bell A, et al. Validation and reproducibility of a high-resolution three-dimensional facial imaging system. Br J Oral Maxillofac Surg 2008;46:27-32. [PubMed]
- Lübbers HT, Medinger L, Kruse A, et al. Precision and accuracy of the 3dMD photogrammetric system in craniomaxillofacial application. J Craniofac Surg 2010;21:763-7. [PubMed]
- Al-Anezi T, Khambay B, Peng MJ, et al. A new method for automatic tracking of facial landmarks in 3D motion captured images (4D). Int J Oral Maxillofac Surg 2013;42:9-18. [PubMed]
- Shujaat S, Khambay BS, Ju X, et al. The clinical application of three-dimensional motion capture (4D): a novel approach to quantify the dynamics of facial animations. Int J Oral Maxillofac Surg 2014;43:907-16. [PubMed]
- Popat H, Richmond S, Playle R, et al. Three-dimensional motion analysis - an exploratory study. Part 1: assessment of facial movement. Orthod Craniofac Res 2008;11:216-23. [PubMed]
- Popat H, Richmond S, Benedikt L, et al. Quantitative analysis of facial movement--a review of three-dimensional imaging techniques. Comput Med Imaging Graph 2009;33:377-83. [PubMed]
- Popat H, Richmond S, Marshall D, et al. Facial movement in 3 dimensions: average templates of lip movement in adults. Otolaryngol Head Neck Surg 2011;145:24-9. [PubMed]
- Wilmore JH, Atwater AE, Maxwell BD, et al. Alterations in breast morphology consequent to a 21-day bust developer program. Med Sci Sports Exerc 1985;17:106-12. [PubMed]
- Kirianoff TG. Volume measurements of unequal breasts. Plast Reconstr Surg 1974;54:616. [PubMed]
- Tegtmeier RE. A quick, accurate mammometer. Ann Plast Surg 1978;1:625-6. [PubMed]
- Ward C, Harrison B. The search for volumetric symmetry in reconstruction of the breast after mastectomy. Br J Plast Surg 1986;39:379-85. [PubMed]
- Wilkie T, Ship AG. Volumetric breast measurement during surgery. Aesthetic Plast Surg 1976;1:301-5. [PubMed]
- Penn J. Breast reduction. Br J Plast Surg 1955;7:357-71. [PubMed]
- Smith DJ Jr, Palin WE Jr, Katch VL, et al. Breast volume and anthropomorphic measurements: normal values. Plast Reconstr Surg 1986;78:331-5. [PubMed]
- Grossman AJ, Roudner LA. A simple means for accurate breast volume determination. Plast Reconstr Surg 1980;66:851-2. [PubMed]
- Rohrich RJ, Hartley W, Brown S. Incidence of breast and chest wall asymmetry in breast augmentation: a retrospective analysis of 100 patients. Plast Reconstr Surg 2006;118:7S-13S; discussion 14S, 15S-17S.
- Jacobs RA, Plastic Surgery Educational Foundation DATA Committee. Three-dimensional photography. Plast Reconstr Surg 2001;107:276-7. [PubMed]
- Harding C, Pompei F, Burmistrov D, et al. Breast Cancer Screening, Incidence, and Mortality Across US Counties. JAMA Intern Med 2015;175:1483-9. [PubMed]
- Da Silveira AC, Daw JL Jr, Kusnoto B, et al. Craniofacial applications of three-dimensional laser surface scanning. J Craniofac Surg 2003;14:449-56. [PubMed]
- Neal AJ, Torr M, Helyer S, et al. Correlation of breast dose heterogeneity with breast size using 3D CT planning and dose-volume histograms. Radiother Oncol 1995;34:210-8. [PubMed]
- Fujii T, Yamaguchi S, Yajima R, et al. Accurate assessment of breast volume by computed tomography using three-dimensional imaging device. Am Surg 2012;78:933-5. [PubMed]
- Fowler PA, Casey CE, Cameron GG, et al. Cyclic changes in composition and volume of the breast during the menstrual cycle, measured by magnetic resonance imaging. Br J Obstet Gynaecol 1990;97:595-602. [PubMed]
- Chae MP, Hunter-Smith DJ, Spychal RT, et al. 3D volumetric analysis for planning breast reconstructive surgery. Breast Cancer Res Treat 2014;146:457-60. [PubMed]
- Chae MP, Rozen WM, McMenamin PG, et al. Emerging Applications of Bedside 3D Printing in Plastic Surgery. Front Surg 2015;2:25. [PubMed]
- Thalmaan D. Die stereogrammetrie: ein diagnostisches hilfsmittel in der kieferorthopaedie (Stereophotogrammetry: a diagnostic device in orthodontology). Zurich, Switzerland: University of Zurich, 1944.
- Tanner JM, Weiner JS. The reliability of the photogrammetric method of anthropometry, with a description of a miniature camera technique. Am J Phys Anthropol 1949;7:145-86. [PubMed]
- Burke PH, Beard FH. Stereophotogrammetry of the face. A preliminary investigation into the accuracy of a simplified system evolved for contour mapping by photography. Am J Orthod 1967;53:769-82. [PubMed]
- Beard LF, Burke PH. Evolution of a system of stereophotogrammetry for the study of facial morphology. Med Biol Illus 1967;17:20-5. [PubMed]
- Burke P. Serial stereophotogrammetric measurements of the soft tissues of the face. A case of a girl with mild facial asymmetry from 3 weeks to 10 years of age. Br Dent J 1983;155:373-9. [PubMed]
- Burke P. Four-dimensional facial change. Br J Orthod 1984;11:170-84. [PubMed]
- Ferrario VF, Sforza C, Dellavia C, et al. Three-dimensional nasal morphology in cleft lip and palate operated adult patients. Ann Plast Surg 2003;51:390-7. [PubMed]
- Nahabedian MY, Galdino G. Symmetrical breast reconstruction: is there a role for three-dimensional digital photography? Plast Reconstr Surg 2003;112:1582-90. [PubMed]
- Neely JG, Cheung JY, Wood M, et al. Computerized quantitative dynamic analysis of facial motion in the paralyzed and synkinetic face. Am J Otol 1992;13:97-107. [PubMed]
- Inokuchi I, Sato K, Ozaki Y. Range-imaging system for 3-D range imaging. 7th ICPR Proceeding. Montreal, Canada, 1984:806.
- Meier-Gallati V, Scriba H, Fisch U. Objective scaling of facial nerve function based on area analysis (OSCAR). Otolaryngol Head Neck Surg 1998;118:545-50. [PubMed]
- Moss JP, Coombes AM, Linney AD, et al. Methods of three dimensional analysis of patients with asymmetry of the face. Proc Finn Dent Soc 1991;87:139-49. [PubMed]
- Esme DL, Bucksch A, Beekman WH. Three-dimensional laser imaging as a valuable tool for specifying changes in breast shape after augmentation mammaplasty. Aesthetic Plast Surg 2009;33:191-5. [PubMed]
- Bush K, Antonyshyn O. Three-dimensional facial anthropometry using a laser surface scanner: validation of the technique. Plast Reconstr Surg 1996;98:226-35. [PubMed]
- Moss JP, Grindrod SR, Linney AD, et al. A computer system for the interactive planning and prediction of maxillofacial surgery. Am J Orthod Dentofacial Orthop 1988;94:469-75. [PubMed]
- Bajaj-Luthra A, Mueller T, Johnson PC. Quantitative analysis of facial motion components: anatomic and nonanatomic motion in normal persons and in patients with complete facial paralysis. Plast Reconstr Surg 1997;99:1894-902; discussion 1903-4.
- Ferrario VF, Sforza C, Poggio CE, et al. Distance from symmetry: a three-dimensional evaluation of facial asymmetry. J Oral Maxillofac Surg 1994;52:1126-32. [PubMed]
- Trotman CA, Gross MM, Moffatt K. Reliability of a three-dimensional method for measuring facial animation: a case report. Angle Orthod 1996;66:195-8. [PubMed]
- Frey M, Giovanoli P, Gerber H, et al. Three-dimensional video analysis of facial movements: a new method to assess the quantity and quality of the smile. Plast Reconstr Surg 1999;104:2032-9. [PubMed]
- Takasaki H. Moiré topography. Appl Opt 1970;9:1467-72. [PubMed]
- Hajeer MY, Ayoub AF, Millett DT, et al. Three-dimensional imaging in orthognathic surgery: the clinical application of a new method. Int J Adult Orthodon Orthognath Surg 2002;17:318-30. [PubMed]
- Olesen OV, Paulsen RR, Højgaar L, et al. Motion tracking in narrow spaces: a structured light approach. Med Image Comput Comput Assist Interv 2010;13:253-60. [PubMed]
- Lane C, Harrell W Jr. Completing the 3-dimensional picture. Am J Orthod Dentofacial Orthop 2008;133:612-20. [PubMed]
- Tepper OM, Unger JG, Small KH, et al. Mammometrics: the standardization of aesthetic and reconstructive breast surgery. Plast Reconstr Surg 2010;125:393-400. [PubMed]
- Tepper OM, Small K, Rudolph L, et al. Virtual 3-dimensional modeling as a valuable adjunct to aesthetic and reconstructive breast surgery. Am J Surg 2006;192:548-51. [PubMed]
- Eder M, Klöppel M, Müller D, et al. 3-D analysis of breast morphology changes after inverted T-scar and vertical-scar reduction mammaplasty over 12 months. J Plast Reconstr Aesthet Surg 2013;66:776-86. [PubMed]
- Eder M, v Waldenfels F, Sichtermann M, et al. Three-dimensional evaluation of breast contour and volume changes following subpectoral augmentation mammaplasty over 6 months. J Plast Reconstr Aesthet Surg 2011;64:1152-60. [PubMed]
- Kovacs L, Eder M, Papadopulos NA, et al. Validating 3-dimensional imaging of the breast. Ann Plast Surg 2005;55:695-6. [PubMed]
- Kovacs L, Eder M, Zimmermann A, et al. Three-dimensional evaluation of breast augmentation and the influence of anatomic and round implants on operative breast shape changes. Aesthetic Plast Surg 2012;36:879-87. [PubMed]
- Tepper OM, Choi M, Small K, et al. An innovative three-dimensional approach to defining the anatomical changes occurring after short scar-medial pedicle reduction mammaplasty. Plast Reconstr Surg 2008;121:1875-85. [PubMed]
- Tepper OM, Small KH, Unger JG, et al. 3D analysis of breast augmentation defines operative changes and their relationship to implant dimensions. Ann Plast Surg 2009;62:570-5. [PubMed]
- Kau CH, Zhurov A, Scheer R, et al. The feasibility of measuring three-dimensional facial morphology in children. Orthod Craniofac Res 2004;7:198-204. [PubMed]
- Geng J. Structured-light 3D surface imaging: a tutorial. Adv Opt Phot 2011;3:128-60.
- Zha XP, Du F, Gao JH, et al. Three-dimensional breast non-contact measurement. Di Yi Jun Yi Da Xue Xue Bao 2005;25:262-6. [PubMed]
- Daly SE, Kent JC, Huynh DQ, et al. The determination of short-term breast volume changes and the rate of synthesis of human milk using computerized breast measurement. Exp Physiol 1992;77:79-87. [PubMed]
- Malata CM, Boot JC, Bradbury ET, et al. Congenital breast asymmetry: subjective and objective assessment. Br J Plast Surg 1994;47:95-102. [PubMed]
- Creasman CN, Mordaunt D, Liolios T, et al. Four-dimensional breast imaging, part II: clinical implementation and validation of a computer imaging system for breast augmentation planning. Aesthet Surg J 2011;31:925-38. [PubMed]
- Creasman CN, Mordaunt D, Liolios T, et al. Four-dimensional breast imaging, part I: introduction of a technology-driven, evidence-based approach to breast augmentation planning. Aesthet Surg J 2011;31:914-24. [PubMed]
- Gabriel A, Fritzsche S, Creasman C, et al. Incidence of breast and chest wall asymmetries: 4D photography. Aesthet Surg J 2011;31:506-10. [PubMed]
- Halazonetis DJ. Acquisition of 3-dimensional shapes from images. Am J Orthod Dentofacial Orthop 2001;119:556-60. [PubMed]
- Moyer HR, Carlson GW, Styblo TM, et al. Three-dimensional digital evaluation of breast symmetry after breast conservation therapy. J Am Coll Surg 2008;207:227-32. [PubMed]
- Liu C, Ji K, Sun J, et al. Does respiration influence breast volumetric change measurement with the three-dimensional scanning technique? Aesthetic Plast Surg 2014;38:115-9. [PubMed]
- Ji K, Luan J, Liu C, et al. A prospective study of breast dynamic morphological changes after dual-plane augmentation mammaplasty with 3D scanning technique. PLoS One 2014;9:e93010. [PubMed]
- Quan M, Fadl A, Small K, et al. Defining pseudoptosis (bottoming out) 3 years after short-scar medial pedicle breast reduction. Aesthetic Plast Surg 2011;35:357-64. [PubMed]
- Winder RJ, Darvann TA, McKnight W, et al. Technical validation of the Di3D stereophotogrammetry surface imaging system. Br J Oral Maxillofac Surg 2008;46:33-7. [PubMed]
- Fourie Z, Damstra J, Gerrits PO, et al. Evaluation of anthropometric accuracy and reliability using different three-dimensional scanning systems. Forensic Sci Int 2011;207:127-34. [PubMed]
- Losken A, Fishman I, Denson DD, et al. An objective evaluation of breast symmetry and shape differences using 3-dimensional images. Ann Plast Surg 2005;55:571-5. [PubMed]
- Schendel SA, Montgomery K. A. Web-based, integrated simulation system for craniofacial surgical planning. Plast Reconstr Surg 2009;123:1099-106. [PubMed]
- Maal TJ, van Loon B, Plooij JM, et al. Registration of 3-dimensional facial photographs for clinical use. J Oral Maxillofac Surg 2010;68:2391-401. [PubMed]
- Aldridge K, Boyadjiev SA, Capone GT, et al. Precision and error of three-dimensional phenotypic measures acquired from 3dMD photogrammetric images. Am J Med Genet A 2005;138A:247-53. [PubMed]
- Chen ZC, Albdour MN, Lizardo JA, et al. Precision of three-dimensional stereo-photogrammetry (3dMD™) in anthropometry of the auricle and its application in microtia reconstruction. J Plast Reconstr Aesthet Surg 2015;68:622-31. [PubMed]
- Reece GP, Merchant F, Andon J, et al. 3D surface imaging of the human female torso in upright to supine positions. Med Eng Phys 2015;37:375-83. [PubMed]
- Oliveira-Santos T, Baumberger C, Constantinescu M, et al. 3D face reconstruction from 2D pictures: first results of a web-based computer aided system for aesthetic procedures. Ann Biomed Eng 2013;41:952-66. [PubMed]
- Tzou CH, Frey M. Evolution of 3D surface imaging systems in facial plastic surgery. Facial Plast Surg Clin North Am 2011;19:591-602. vii. [PubMed]
- Al-Hiyali A, Ayoub A, Ju X, et al. The Impact of Orthognathic Surgery on Facial Expressions. J Oral Maxillofac Surg 2015;73:2380-90. [PubMed]
- Gerstle TL, Ibrahim AM, Kim PS, et al. A plastic surgery application in evolution: three-dimensional printing. Plast Reconstr Surg 2014;133:446-51. [PubMed]
- Chhaya MP, Melchels FP, Holzapfel BM, et al. Sustained regeneration of high-volume adipose tissue for breast reconstruction using computer aided design and biomanufacturing. Biomaterials 2015;52:551-60. [PubMed]
- Kiarashi N, Nolte AC, Sturgeon GM, et al. Development of realistic physical breast phantoms matched to virtual breast phantoms based on human subject data. Med Phys 2015;42:4116-26. [PubMed]
- Chae MP, Hunter-Smith DJ, Rozen WM. Image-guided 3D-printing and haptic modeling in plastic surgery. In: Saba L, Rozen WM, Alonso-Burgos A, et al, editors. Imaging in plastic surgery. London: CRC Taylor and Francis Press, 2014.
- Ciocca L, De Crescenzio F, Fantini M, et al. Rehabilitation of the nose using CAD/CAM and rapid prototyping technology after ablative surgery of squamous cell carcinoma: a pilot clinical report. Int J Oral Maxillofac Implants 2010;25:808-12. [PubMed]
- Cohen A, Laviv A, Berman P, et al. Mandibular reconstruction using stereolithographic 3-dimensional printing modeling technology. Oral Surg Oral Med Oral Pathol Oral Radiol Endod 2009;108:661-6. [PubMed]
- Chen YX, Zhang K, Hao YN, et al. Research status and application prospects of digital technology in orthopaedics. Orthop Surg 2012;4:131-8. [PubMed]
- Gillis JA, Morris SF. Three-dimensional printing of perforator vascular anatomy. Plast Reconstr Surg 2014;133:80e-2e. [PubMed]
- Way TP, Barner KE. Automatic visual to tactile translation--Part II: Evaluation of the TACTile Image Creation System. IEEE Trans Rehabil Eng 1997;5:95-105. [PubMed]
- Melchels F, Wiggenhauser PS, Warne D, et al. CAD/CAM-assisted breast reconstruction. Biofabrication 2011;3:034114. [PubMed]
- Chae MP, Hunter-Smith DJ, De-Silva I, et al. Four-Dimensional (4D) Printing: A New Evolution in Computed Tomography-Guided Stereolithographic Modeling. Principles and Application. J Reconstr Microsurg 2015;31:458-63. [PubMed]
- Siva S, Chesson B, Callahan JW, et al. Dosimetric Consequences of 3D Versus 4D PET/CT for Target Delineation of Lung Stereotactic Radiotherapy. J Thorac Oncol 2015;10:1112-5. [PubMed]
- Rozen WM, Whitaker IS, Stella DL, et al. The radiation exposure of Computed Tomographic Angiography (CTA) in DIEP flap planning: low dose but high impact. J Plast Reconstr Aesthet Surg 2009;62:e654-5. [PubMed]
- Meattini I, Marrazzo L, Zani M, et al. Four-dimensional computed tomography in accelerated partial breast irradiation planning: single series from a phase III trial. Radiol Med 2015;120:1078-82. [PubMed]
- Siva S, Pham D, Gill S, et al. An analysis of respiratory induced kidney motion on four-dimensional computed tomography and its implications for stereotactic kidney radiotherapy. Radiat Oncol 2013;8:248. [PubMed]
- Huguet F, Yorke ED, Davidson M, et al. Modeling pancreatic tumor motion using 4-dimensional computed tomography and surrogate markers. Int J Radiat Oncol Biol Phys 2015;91:579-87. [PubMed]
- Rozen WM, Chubb D, Crossett M, et al. The future in perforator flap imaging: a new technique to substantially reduce radiation dose with computed tomographic angiography. Plast Reconstr Surg 2010;126:98e-100e. [PubMed]
- Nie JY, Lu LJ, Gong X, et al. Delineating the vascular territory (perforasome) of a perforator in the lower extremity of the rabbit with four-dimensional computed tomographic angiography. Plast Reconstr Surg 2013;131:565-71. [PubMed]
- Colohan S, Wong C, Lakhiani C, et al. The free descending branch muscle-sparing latissimus dorsi flap: vascular anatomy and clinical applications. Plast Reconstr Surg 2012;130:776e-787e. [PubMed]