Current controversies and future directions in the diagnosis and management of differentiated thyroid cancers
Introduction
Thyroid cancer is the most common endocrine gland malignancy worldwide and has increased in incidence over the past few decades (1,2). In fact, thyroid cancer is now the fifth most common cancer diagnosis in women; it is expected to become the second most common malignancy in women and ninth most common in men by the year 2030 (2). Despite the marked increase in incidence, especially of early/smaller lesions, there has been no change in thyroid cancer mortality (1,2). This suggests that we may be over-diagnosing and over-treating thyroid tumors that may never lead to symptoms or death (2). As a result, the latest guidelines for the diagnosis and treatment of thyroid cancers from many international societies have shifted to focus on limiting unnecessary screening and treatment (2-5). The most recent American Thyroid Association (ATA) Management Guidelines for Adult Patients with Thyroid Nodules and Differentiated Cancer—considered the standard for the diagnosis and management of thyroid cancer in the US—were released in 2015 (4). Approximately 70% of these guidelines remain unchanged from the prior version released in 2009; however, there were key changes that were intended to directly alter clinical practice. These changes have resulted in controversy and represent opportunities for future research (2).
It has long been known that differentiated thyroid cancers (DTCs), especially papillary thyroid cancers (PTCs), typically follow a more slow-growing and indolent course than many other malignancies, and historically a large number of these tumors were only diagnosed on autopsy following death by other causes (2,6). Taken together, the increasing incidence of thyroid cancer, especially of smaller tumors, and unchanged mortality have triggered a re-evaluation of the treatment for DTC. Accordingly, for those patients requiring surgical intervention, the extent of surgical resection and whether any adjuvant therapy is necessary remains controversial.
Currently, much of the research on DTC has focused on several key areas where the optimal algorithm for diagnosis and treatment remains unclear: the management of patients with indeterminate cytopathology on fine needle aspirate (FNA); the extent of surgical resection required for those with differentiated cancer; the role of adjuvant radioactive iodine (RAI) therapy; and novel adjuvant therapies for locally advanced or metastatic disease.
Management of indeterminate nodules on FNA cytopathology: role of molecular testing
The Bethesda grading system for thyroid nodule FNAs, introduced in 2007, standardized the reporting of cytopathology results, and successfully and reliably stratifies thyroid nodules based on their risk of malignancy (7,8). Bethesda V lesions carry a 60–75% risk of malignancy, and there is largely a consensus recommendation for surgery for these patients (4,8). However, anywhere from 15–40% of FNA samples are scored either as Bethesda III or IV indeterminate lesions (9). These lesions carry a 15–30% risk of malignancy (8). Originally, repeat biopsy was recommended for Bethesda III lesions and diagnostic hemithyroidectomy was recommended for Bethesda IV lesions (10). However, the majority (70–85%) of surgical specimens from patients who undergo hemithyroidectomy for Bethesda III and IV lesions are ultimately benign, therefore resulting in seemingly non-therapeutic surgeries (11). Furthermore, hemithyroidectomy is not without consequences as up to 43% of patients will develop hypothyroidism requiring lifelong thyroid hormone supplementation (12).
Consequently, much research has been focused on further characterizing these Bethesda III and IV indeterminate lesions to better identify patients at risk for underlying malignancy and to avoid unnecessary surgery in those with benign nodules. Although there is evidence that specific cytopathologic features of these lesions that may indicate a higher risk of malignancy (13), the 2015 ATA guidelines focus on molecular testing as a novel supplemental diagnostic tool (4). This is in large part due to recent data that demonstrates that certain genetic alterations, such as BRAFV600E and RAS mutations, and rearranged during transfection (RET)/PTC and PAX8/peroxisome proliferator-activated receptor gamma-1 (PPARγ) translocations, have been shown to be predictive of malignancy (14-25). Additional progress has been made using gene expression of mRNA and microRNA to predict malignancy, and these assays have also been shown to improve our ability to distinguish malignant and benign lesions using FNA.
Genetic alterations: BRAFV600E, RAS, RET/PTC, and PAX8/PPARγ
B-type RAF kinase is a serine-threonine kinase involved in the RAS-MAPK cell signaling cascade that is activated by phosphorylation of Thr598 and Ser601 (19,20,25). In approximately 40% of PTCs, a mutant form of the protein with glutamine substituted for valine at residue 600 (BRAFV600E) mimics the activated wild-type protein and triggers constitutive phosphorylation of ERK1/2 and alteration of associated transcription factors leading to malignancy (20). Multiple studies have demonstrated association between BRAFV600E mutation and a more aggressive phenotype; a recent meta-analysis demonstrated that the mutation was associated with an increased rate of lymph node metastasis, extrathyroidal extension, resistance to RAI therapy, and even decreased overall survival (26). Other studies have shown a decreased disease-free survival (27). However, it remains to be seen whether preoperative knowledge of BRAF mutation status will affect operative planning and decision making (28). Regardless, the mutation is diagnostic of PTC, and can be used as an adjunct test in otherwise indeterminate thyroid FNA samples (19,20). BRAFV600E testing is presently included in many commercially available gene-based tools for diagnosis of thyroid cancer.
The second most common group of genetic mutations in DTCs involve the RAS family of proteins, specifically KRAS, NRAS, or HRAS (22). Mutations in the RAS genes are found in less than 5% of all thyroid nodules (29), but are detectable in FNA specimens (23), and when present are nearly always associated with a follicular cytologic pattern (30) (see Table 1). RAS mutations are found in benign follicular neoplasms, and tumors with isolated RAS mutations are usually associated with less aggressive features and a more indolent course (23). However, despite their occurrence in benign lesions, a recent meta-analysis demonstrated that RAS mutations have a 93% specificity for malignancy (22). This has not translated to a reliable single-gene marker for malignancy, as the positive and negative predictive values (PPV and NPV) in the same analysis were only 78% and 64%, respectively (22). Still, when combined with analysis of other genetic mutations, the RAS family of genes has become a useful data point in clarifying the risk of malignancy of indeterminate lesions. In fact, there is evidence that the risk of malignancy varies with the form of RAS that is mutated. One group demonstrated that the specific isoform of RAS that was mutated in follicular neoplasms determined the relative risk of malignancy, with 100% of KRAS mutant tumors being malignant, as compared to 74% for NRAS, and 56% for HRAS (18).
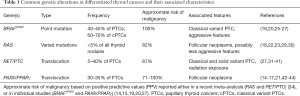
Full table
In addition to genetic mutations, certain translocations have also been identified. The most common translocations known to occur in PTCs involve the RET gene, which codes for a tyrosine kinase that is normally not expressed in thyroid tissues (31,32). There are at least 13 different RET translocations identified in PTCs to date, and all are thought to trigger activation of the MAPK pathway leading to malignancy (33). A meta-analysis by Rodrigues et al. in 2012 found presence of a RET/PTC translocation had a 87% PPV for malignancy (34). The two most common translocations, RET/PTC1 and RET/PTC3, have both been shown to be strongly associated with PTCs, and are thought to be nearly mutually exclusive with BRAF and RAS mutant tumors (32,33,35). Tumors with the RET/PTC3 translocation are noted to more commonly be solid variant PTC and occur more frequently in patients with a history of radiation exposure (33,36-38). Earlier studies seemed to show an association between RET/PTC translocations, especially RET/PTC1 translocations, and a favorable prognosis (39-41). Recently, however, several studies have noted an association between RET/PTC3 translocation and distant metastases (27,36), and patients with RET/PTC positive tumors have been shown to have a disease pattern similar to patients with the BRAFV600E mutation (27). As our understanding of the prognostic impact of RET/PTC translocations becomes clearer, we expect that the marker will be increasingly important in the management of PTC.
PAX8/PPARγ translocations are the second most common translocations in thyroid cancers, and involve the fusion of the PPARγ gene with the thyroid specific transcription factor PAX8 (21). These translocations are found in up to 35% of follicular thyroid cancers, but also found in 2–13% of follicular adenomas and in 1–5% of PTCs—nearly always follicular variant (15-17,42,43). In a recent retrospective analysis by Armstrong et al., 1.1% of PTCs were found to have this translocation. 16 patients were identified on pre-operative FNA, all of them were diagnosed as either benign or indeterminate lesions on FNA, however all were subsequently diagnosed with cancer; a 100% PPV (14). However, the translocation occurs with variable frequency in benign adenomas, obscuring it’s utility as a single molecular marker of malignancy (44).
Genetic testing: ThyroSeq® and ThyGenX®
In the US, these four genes have been combined with other, less common genetic alterations to form the basis of commercially available next generation sequencing assays. The developers of the ThyroSeq® assay demonstrated that the most recent iteration of the test, ThyroSeq® v.2, had a PPV of 77% in a population with a 28% incidence of malignancy in Bethesda IV lesions (45). However, as the authors note, there is still room for substantial improvement. Similarly, ThyGenX® is a seven-gene mutation panel that has been shown to have PPV and NPV of 71% and 85%, respectively. ThyGenX® is combined with ThyraMIR™, a reflex microRNA based assay run on patient samples with an indeterminate ThyGenX® result (46) (see Table 2).
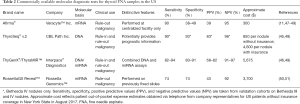
Full table
Gene expression alterations: Afirma®
The Afirma® gene expression classifier is a proprietary gene expression assay first made publicly available in the US in 2010 by Veracyte™ Inc. In centers that perform Afirma® testing, two samples are often collected at the time of FNA, one for cytopathological examination, and another for Afirma®. The Afirma® vial is discarded if the cytopathology is diagnostic of either a benign or malignant lesion. If the lesion is indeterminate, RNA is isolated from the second sample and tested using a proprietary microarray assay (47). The assay is designed to classify nodules found to be indeterminate on traditional cytology reports as either “benign” (>95% NPV) or “suspicious” (approximately 40% risk of malignancy) based on the RNA expression pattern (11,47). Use of Afirma® testing has been shown to dramatically decrease the rate of diagnostic thyroidectomy and thyroid lobectomy; one study found that for every two Afirma® tests run on indeterminate nodules one surgery was avoided (11). However, in a large validation study by Alexander et al. in 2012, the PPV of Afirma® testing was found to be only 38% (48). Therefore, Afirma® may be a good “rule out” test for malignancy when the result is negative, but a positive result can still lead many patients to undergo a potentially unnecessary operation.
MicroRNA alterations
MicroRNAs, or miRNAs, are small (usually 20–30 nucleotides), non-coding, regulatory RNA molecules which downregulate expression of target messenger RNAs (52). These molecules are differentially expressed in malignancy, and numerous studies have shown that malignant and benign thyroid tissues have differential miRNA signatures (52-56). Our group identified four such miRNAs (miR-222, miR-21, miR-181a, and miR-146b) that are differentially expressed in PTC, and prospectively demonstrated that these four miRNAs can be used to predict malignancy in indeterminate thyroid nodules with 100% sensitivity and 86% specificity (56). Others have suggested miRNA panels consisting of upwards of 15 miRNAs could be used to diagnose cancer in indeterminate cytology, although that model has yet to be tested prospectively on a large scale (57).
Presently, there are commercially available miRNA assays for thyroid cancer available in the US, and two have been recently validated for diagnostic use: ThyGenX®/ThyraMIR™ and RosettaGX Reveal™. ThyraMIR™ is only offered in combination with ThyGenX®. It assesses levels of 10 miRNAs and has been shown to have an independent sensitivity of 57% and specificity of 92%. When combined with the DNA-based ThyGenX® assay, the test achieved 89% sensitivity and 85% specificity, with 74% and 94% PPV and NPV, respectively (46). The RosettaGX Reveal™ assay is unique in that it is designed to be performed on FNA slides, so that it can be ordered as an additional test even after cytopathology results have returned, without the need for additional tissue samples (50). Using 24 miRNAs, the creators of the assay were able to demonstrate a >95% sensitivity and >70% specificity (depending on number of available thyrocytes) on 106 cytopathology specimens (51). These tests are expensive—often thousands of US dollars (49) (Table 2), but may be of increasing clinical importance as recommendations for surgical management of thyroid nodules continues to evolve.
Ultimately, the PPV and NPV of any molecular test is highly dependent on the pre-test probability of cancer. These assays are imperfect tests, but they have shown benefit in detecting malignancy and reducing unnecessary surgery for patients with thyroid nodules (11,45,48,58,59) (see Table 2).
Extent of surgery for differentiated tumors
Many of the changes in the 2015 ATA guidelines involve recommendations regarding the surgical management of patients with biopsy proven DTC. These include a new recommendation for preoperative cross-sectional imaging for patients with clinically advanced disease, and include hemithyroidectomy as an acceptable option for malignant nodules 1–4 cm in diameter (2).
Previously, complete neck ultrasound was considered sufficient for preoperative staging prior to planned thyroidectomy for cancer (2,4). However, the latest ATA guidelines recommend cross-sectional imaging—preferably CT scan with IV contrast—as an adjunct to complete neck ultrasound to improve identification of cervical lymphadenopathy for patients with “clinical suspicion of advanced disease”, such as palpable regional lymphadenopathy (4). Additionally, the ATA no longer recommends adjuvant treatment solely for lymph node micrometastases (<0.2 cm, less than 5 involved nodes). It is now known that micronodal metastases are common in thyroid cancer but have little to no effect on long-term prognosis (60). This has increased the importance of preoperative imaging and staging in order to better detect macrometastases (>0.2 cm) to prevent under-staging. Recently, multiple studies have demonstrated improved preoperative staging with the addition of CT scans (61-63). There remains little evidence for the benefit of performing prophylactic central neck lymph node dissection in cases where both ultrasound and CT show no evidence of lymph node involvement (4). Thus, preoperative CT scans may prevent unnecessary prophylactic central neck dissections. The current ATA recommendations suggest prophylactic central neck dissection only in cases of papillary carcinoma where the tumor is either T3 or T4 or there is clinical evidence of lateral neck lymphadenopathy (4). Follicular cancers are considered lower risk for lymph node metastasis and thus these guidelines are largely addressing PTCs (64,65).
Perhaps the most controversial change in the 2015 recommendations is the addition of language that suggests that hemithyroidectomy is equivalent to total thyroidectomy for low risk cancers between 1–4 cm in diameter (2). The previous recommendation for total thyroidectomy for these patients was based partially on retrospective studies demonstrating improved survival (66) and decreased local recurrence rates (67,68) for patients undergoing total thyroidectomy. Bilimoria et al. examined 52,173 patients in the National Cancer Database that underwent either total thyroidectomy or lobectomy for PTC from 1985–1998 and found a higher risk of both recurrence and death in the lobectomy group for patients with tumors >1 cm (66). However, data were not available to determine how many of these patients in the lobectomy group had high risk features that would have made them better candidates for total thyroidectomy. A follow-up study by Adam et al. in 2014 analyzed 61,775 patients from the same database undergoing thyroidectomy or lobectomy from 1998 to 2006. When adjusted for high risk features such as extrathyroidal extension and nodal or multifocal disease, a multivariate analysis showed equivalent overall survival between the two groups (69). Furthermore, multiple recent studies have offered additional evidence that for low risk tumors between 1–4 cm, lobectomy may be equivalent to total thyroidectomy for the treatment of PTC (70-74).
This recommendation has already been called into question, however. One of the major concerns regarding the equivalence of lobectomy and thyroidectomy for low risk tumors is the fear that many of these patients will need a second surgery for completion thyroidectomy. This concern stems from the fact that some low risk patients will ultimately have microscopic high-risk features on final pathology; these patients are recommended to undergo adjuvant treatment with RAI that can only be given after completion thyroidectomy. Kluijfhout et al. retrospectively analyzed 394 patients with ATA low or low-to-intermediate risk DTCs from 1–4 cm who underwent either a hemi or total thyroidectomy from 2000–2010 at their institution. They found that 25.6% of the patients who underwent hemithyroidectomy would ultimately benefit from RAI per the ATA guidelines (75).
Further criticism of the recommendation has been centered on the risk of contralateral malignancy that would be left behind with hemithyroidectomy. Historically, studies have demonstrated that up to 50% of patients with PTC have bilateral cancers (76,77). A recent retrospective analysis by Kim et al. found 29.4% of patients with classical type PTC between 1–4 cm had contralateral cancers at the time of surgery. Importantly, patients who underwent only hemithyroidectomy were excluded from the study, and the authors do not state how many, if any, of these patients would have been eligible for hemithyroidectomy under the ATA guidelines (78). Notably, many of the features denoted as high risk by the ATA are strongly associated with multifocality (77,79-81); suggesting that these patients would have undergone total thyroidectomy under the current guidelines.
In light of these findings, our practice is to discuss elective total thyroidectomy with patients who have contralateral nodules on preoperative imaging. Those patients who elect to undergo hemithyroidectomy are educated about the possible need for a completion thyroidectomy should there be aggressive features on final pathology. Additionally, preoperative consent is obtained for total thyroidectomy in the event that advanced disease is discovered intraoperatively.
Adjuvant RAI therapy
RAI therapy for patients at moderate risk of recurrence
RAI therapy has been shown to reduce recurrence rates and improve disease free survival in patients with locally advanced or even metastatic disease (82-84) and has been in use to treat thyroid cancers in the US since the mid-1940s (85). RAI was originally recommended for all patients with DTC, but it is associated with potentially permanent side effects, including salivary, lacrimal, and gonadal dysfunction, and risk of secondary malignancy (86-88). Therefore, much research and debate over the past 5–10 years has focused on identifying those patients who stand to benefit the most from RAI. The most recent guidelines from the Society of Nuclear Medicine and Molecular Imaging [2012] and from the British Thyroid Association [2014] continue to recommend consideration of RAI for all patients with DTCs greater than 1 cm in diameter (89,90). In contrast, the latest ATA guidelines recommend adjuvant RAI therapy only for patients with either intermediate or high risk of recurrence (according to the ATA Risk of Recurrence Stratification) after total thyroidectomy (4). Similarly, the European Thyroid Association (ETA) guidelines separate patients into very-low-risk, low-risk, and high-risk patients, recommending RAI for the high-risk group only; this latter group represents patients that would be separated into intermediate and high risk under the ATA guidelines. These recommendations are based on an increasing volume of research that demonstrates that patients with low-risk tumors do not receive any benefit from RAI (5). However, there remains controversy over which patients of intermediate risk benefit from adjuvant RAI therapy.
The recommendation to continue RAI for patients with intermediate risk is based on recent studies demonstrating a small, yet statistically significant, difference in overall survival for patients in the intermediate risk group (4,91-93). Ruel et al. examined 21,870 patients in the SEER database that met ATA criteria for intermediate risk DTC and found improved overall survival for those who received post-operative RAI treatment. These authors noted a 29% overall reduction in the risk of death for patients treated with RAI, and importantly a 36% reduction for patients younger than age 45 years (91). A more recent analysis by Zhang et al. in 2017 confirmed the OS benefit from RAI therapy; however, these authors also analyzed disease specific survival and found no benefit. They concluded, therefore, that patients with intermediate risk cancers might not benefit from RAI therapy (94). Similarly, in a recent review of almost nine thousand South Korean patients from 1997–2015, Kim et al. demonstrated that there was no decrease in loco-regional recurrence for patients undergoing RAI, even in patients with BRAFV600E positive, multifocal, or larger (>1 cm) tumors, or those with regional lymph node metastasis or extrathyroidal extension (95).
RAI dosing for remnant ablation
RAI following thyroidectomy is used for three distinct primary clinical goals: (I) to ablate remnant thyroid tissue in order to facilitate monitoring for recurrence after thyroidectomy; (II) as adjuvant therapy to decrease the likelihood of locoregional recurrence or distant metastasis; and (III) as therapy for residual, unresectable primary or metastatic disease (4). Different doses are currently recommended for each of these indications, and recent trends have been towards decreasing the dose of RAI, especially for remnant ablation (5). Mallick et al. found that lower doses of I131 were equally effective at successful remnant ablation post-thyroidectomy as higher doses and reduced the rate of adverse events (96). Similarly, Schlumberger et al. demonstrated that for low-risk tumors, low dose RAI was effective and sufficient for remnant ablation (97). However, other studies have found contradictory results: one group found that lower I131 doses were not as successful at remnant ablation, although higher doses were associated with more adverse events (98). Another study noted that patients receiving a lower initial dose more frequently needed a second dose to achieve successful ablation (99). While the ATA guidelines have sided with studies demonstrating that lower RAI doses may be as effective as higher doses with less adverse events, there remains disagreement in the literature, and further randomized trials are necessary to better identify those patients who may be safely treated with a lower dose.
Adjuvant therapies for RAI-resistant and/or locally advanced and metastatic disease
Advanced and metastatic DTCs show minimal response to traditional cytotoxic chemotherapies (100-102), historically leaving clinicians few options for adjuvant therapy, especially for RAI-resistant disease. Recently, however, novel drugs known as tyrosine kinase inhibitors (TKIs) have been approved for the treatment of advanced DTCs (103,104). These drugs are non-specific inhibitors of various proteins in the MAPK pathway, the principal pathway activated in many DTCs (105). Many of these drugs have activity against BRAF, RET/PTC, and other targets downstream of the RAS proteins, and they have shown promise in slowing disease progression for patients with advanced cancers. Lenvatinib and sorafenib are the two TKIs currently approved by the US Food and Drug Administration for use in DTC, but others, including cabozantinib and vandetanib have been used to treat DTC as well (105-109). However, resistance to these drugs can develop quickly (110-112), and their use is limited by occasionally severe adverse events (113).
Presently, lenvatinib is increasingly being used as first line therapy for patients with advanced RAI-resistant DTC (104,105,114). The SELECT Trial, a phase III, multi-institutional trial, compared treatment of patients with advanced, RAI-resistant DTC with placebo and assessed progression free survival, overall survival, response rate, and safety. Median progression free survival was five times greater in the treatment group (18.3 compared to 3.6 months) with a response rate of 64.8%. The endpoint of overall survival was not reached. Adverse events were common, with greater than 97% of the treatment group experiencing some side effects (most commonly hypertension, diarrhea, fatigue, and anorexia and weight loss), but they were typically not severe enough to limit treatment (115). This trial was one of the most recent in a series of randomized trials that demonstrated a dramatic effect of lenvatinib in DTC, and led to its approval by the FDA.
As TKI use becomes more common in thyroid cancer, resistance to the drugs may become a more clinically important issue in the future. Resistance to TKIs has been demonstrated in a variety of other cancers, including non-small cell lung cancers, leukemias, melanomas, gliomas, and others (111,116-118). Compared to their use in other cancers, TKI use for thyroid cancer is relatively new, and there are correspondingly few studies addressing resistance to these drugs in DTC. A recent review of the mechanisms of resistance to TKIs in melanoma by Johnson et al. noted that data suggests that the primary mechanism of acquired resistance to these drugs is escape via related but alternate kinase pathways that become activated upon blockade of the target pathway. This mechanism, termed “adaptive kinase reprogramming” can be combatted by combining TKIs to target additional proteins, with the goal of either restored efficacy or delayed onset of resistance (111). Beadnell et al. demonstrated that thyroid cancer cells can acquire resistance to dasatinib in vitro via activation of the MAPK pathway, and that the addition of a MEK1/2 inhibitor restored sensitivity (119). These studies suggest that in the future, drug cocktails may become necessary for very high-risk patients.
Finally, TKIs may be able to restore RAI sensitivity in select patients. The BRAFV600E mutation has been shown to alter sodium-iodide symporter (NIS) expression and cytologic location and decrease response to RAI therapy in some patients (25,120-122). Gao et al. performed a retrospective review of 109 patients with classical PTC and found that BRAFV600E mutation was associated with significantly decreased NIS expression (120). Yang et al. reviewed 73 PTC patients with distant metastases and found that patients with BRAFV600E mutations were much more likely to have non-iodine avid metastases: 84.2% compared to only 5.6% of patients without the mutation (123). Our group showed that BRAF silencing with siRNA coupled with TSH stimulation induced TSH receptor and NIS expression in vitro in a BRAFV600E mutant thyroid cell line (121). Other studies have demonstrated that TKIs can increase or restore NIS expression and/or RAI sensitivity in patients and mice with DTC (122,124,125). Ho et al. treated RAI resistant patients with selumetinib, an MEK1/2 inhibitor, and were able to restore RAI uptake (as measured by I124 PET) in 12/20 patients; 4/9 with BRAF mutations and 5/5 with NRAS mutations. Eight of these 12 reached the uptake threshold for treatment with I131, with 5/8 achieving partial response and the remaining three with stable disease (122). Although this is a small study, it illustrates the potential for the use of TKIs to restore RAI sensitivity or increase its effectiveness in patients with mutations in the MAPK pathway, see Figure 1.
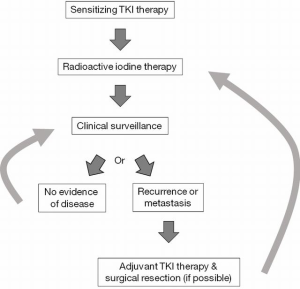
Conclusions and future directions
DTC is becoming increasingly common, yet fortunately, few people will die of the disease. Recent changes in the management of thyroid cancer have focused on de-escalating care. For example, the ATA no longer recommends biopsy of thyroid lesions smaller than 1cm, even those with worrisome features on ultrasound imaging (4). One type of tumor that was previously considered malignant, encapsulated follicular-variant PTC, is now classified as benign noninvasive follicular thyroid neoplasm with papillary-like nuclear features (NIFTP), based on the excellent prognosis for these patients (126). Identifying those patients with aggressive disease becomes increasingly important as clinicians continue to advocate for fewer and less aggressive interventions for patients with DTCs.
Molecular markers offer promise for the diagnosis of malignant lesions on FNA biopsy, and potentially will help to differentiate aggressive and indolent disease in the future. It has been well documented that tumors with BRAFV600E mutations tend to be more aggressive, but that knowledge has yet to affect clinical decision making on a large scale. Similarly, RET/PTC translocations may portend a more aggressive clinical course and offer a potential therapeutic target. There are already commercially available TKIs with RET targeting activity, such as cabozantinib, but these are also not yet approved for use in thyroid cancer.
RAI therapy offers a unique treatment option for DTC patients, but even after decades of use, it remains unclear which patients benefit most and what the optimal dosing regimen is for ablative therapy. Randomized trials have shown mixed results, and many have raised concerns that lower doses of RAI may be ineffective or insufficient. In order to determine optimal dosing and treatment regimens of RAI, a large, multicenter randomized trial with long-term follow-up is required. Until such data becomes available, clinicians must base their decisions on conflicting data from smaller studies in variable patient populations.
Targeted TKI therapy may offer new hope in terms of prolonging disease-free survival for those rare patients with RAI resistant and/or unresectable disease. Lenvatinib has shown promise in treating patients with thyroid cancer, and other drugs might soon gain FDA approval in the US. In the future, genetic analysis might allow targeting to each patient’s tumor specific genetic profile—for example, treating BRAF mutant tumors with dabrafenib or RET/PTC translocated tumors with cabozantinib. However, as these treatments become more widely available and more frequently used, we may find more patients with tumors that become resistant to TKIs. Furthermore, while many patients demonstrate partial response to TKI treatment, very few are clinically cured of their disease. These drugs potentially have an additional benefit that is unique to thyroid cancer, that is, restoring RAI sensitivity in otherwise resistant tumors. Selumetinib has been shown to potentially increase or restore RAI sensitivity (122), and larger-scale studies are currently underway using other TKIs hoping to do the same.
Ultimately, the future of the diagnosis and treatment of thyroid cancer lies in differentiating those aggressive tumors that will lead to clinically persistent or metastatic disease from those that will not. As our understanding of the molecular biology and biochemistry of these cancers improves, perhaps more tumor types will follow the path of encapsulated follicular-variant PTC and begin to be treated as benign lesions. It is with this in mind that many have advocated for fewer screening ultrasounds and biopsies and less aggressive treatment for DTCs. However, in order for that trend to safely continue, we must demonstrate that not only can we reliably and repeatedly identify aggressive tumor subtypes, but that we also have effective, targeted treatments to offer those patients in the event that decreased screening delays diagnosis. This pendulum between over- and under-treatment is what drives much of the controversy surrounding the current management of thyroid cancer, and will continue to be the focus of future research.
Acknowledgements
None.
Footnote
Conflicts of Interest: The authors have no conflicts of interest to declare.
References
- Davies L, Welch HG. Current thyroid cancer trends in the United States. JAMA Otolaryngol Head Neck Surg 2014;140:317-22. [Crossref] [PubMed]
- Haugen BR. 2015 American Thyroid Association Management Guidelines for Adult Patients with Thyroid Nodules and Differentiated Thyroid Cancer: What is new and what has changed? Cancer 2017;123:372-81. [Crossref] [PubMed]
- Leenhardt L, Erdogan MF, Hegedus L, et al. 2013 European thyroid association guidelines for cervical ultrasound scan and ultrasound-guided techniques in the postoperative management of patients with thyroid cancer. Eur Thyroid J 2013;2:147-59. [Crossref] [PubMed]
- Haugen BR, Alexander EK, Bible KC, et al. 2015 American Thyroid Association Management Guidelines for Adult Patients with Thyroid Nodules and Differentiated Thyroid Cancer: The American Thyroid Association Guidelines Task Force on Thyroid Nodules and Differentiated Thyroid Cancer. Thyroid 2016;26:1-133. [Crossref] [PubMed]
- Marti JL, Morris LG, Ho AS. Selective use of radioactive iodine (RAI) in thyroid cancer: No longer “one size fits all”. Eur J Surg Oncol 2018;44:348-56. [Crossref] [PubMed]
- Vanderlaan WP. The occurrence of carcinoma of the thyroid gland in autopsy material. N Engl J Med 1947;237:221. [Crossref] [PubMed]
- Baloch ZW, LiVolsi VA, Asa SL, et al. Diagnostic terminology and morphologic criteria for cytologic diagnosis of thyroid lesions: a synopsis of the National Cancer Institute Thyroid Fine-Needle Aspiration State of the Science Conference. Diagn Cytopathol 2008;36:425-37.
- Wesoła M, Jeleń M. Bethesda System in the evaluation of thyroid nodules Adv Clin Exp Med 2017;26:177-82. Review. [Crossref] [PubMed]
- Park HJ, Moon JH, Yom CK, et al. Thyroid “atypia of undetermined significance” with nuclear atypia has high rates of malignancy and BRAF mutation. Cancer Cytopathol 2014;122:512-20. [Crossref] [PubMed]
- Renuka IV, Saila Bala G, Aparna C, et al. The bethesda system for reporting thyroid cytopathology: interpretation and guidelines in surgical treatment. Indian J Otolaryngol Head Neck Surg 2012;64:305-11. [Crossref] [PubMed]
- Duick DS, Klopper JP, Diggans JC, et al. The impact of benign gene expression classifier test results on the endocrinologist-patient decision to operate on patients with thyroid nodules with indeterminate fine-needle aspiration cytopathology. Thyroid 2012;22:996-1001. [Crossref] [PubMed]
- Chu KK, Lang BH. Clinicopathologic predictors for early and late biochemical hypothyroidism after hemithyroidectomy. Am J Surg 2012;203:461-6. [Crossref] [PubMed]
- Gan TR, Nga ME, Lum JH, et al. Thyroid cytology-nuclear versus architectural atypia within the “Atypia of undetermined significance/follicular lesion of undetermined significance” Bethesda category have significantly different rates of malignancy. Cancer Cytopathol 2017;125:245-56. [PubMed]
- Armstrong MJ, Yang H, Yip L, et al. PAX8/PPARγ rearrangement in thyroid nodules predicts follicular-pattern carcinomas, in particular the encapsulated follicular variant of papillary carcinoma. Thyroid 2014;24:1369-74. [Crossref] [PubMed]
- Marques AR, Espadinha C, Catarino AL, et al. Expression of PAX8-PPAR gamma 1 rearrangements in both follicular thyroid carcinomas and adenomas. J Clin Endocrinol Metab 2002;87:3947-52. [PubMed]
- Nikiforova MN, Lynch RA, Biddinger PW, et al. RAS point mutations and PAX8-PPAR gamma rearrangement in thyroid tumors: evidence for distinct molecular pathways in thyroid follicular carcinoma. J Clin Endocrinol Metab 2003;88:2318-26. [Crossref] [PubMed]
- Dwight T, Thoppe SR, Foukakis T, et al. Involvement of the PAX8/peroxisome proliferator-activated receptor gamma rearrangement in follicular thyroid tumors. J Clin Endocrinol Metab 2003;88:4440-5. [Crossref] [PubMed]
- Fukahori M, Yoshida A, Hayashi H, et al. The associations between RAS mutations and clinical characteristics in follicular thyroid tumors: new insights from a single center and a large patient cohort. Thyroid 2012;22:683-9. [Crossref] [PubMed]
- Ohori NP, Singhal R, Nikiforova MN, et al. BRAF mutation detection in indeterminate thyroid cytology specimens: underlying cytologic, molecular, and pathologic characteristics of papillary thyroid carcinoma. Cancer Cytopathol 2013;121:197-205. [Crossref] [PubMed]
- Li X, Abdel-Mageed AB, Kandil E. BRAF mutation in papillary thyroid carcinoma. Int J Clin Exp Med 2012;5:310-5. [PubMed]
- Kroll TG, Sarraf P, Pecciarini L, et al. PAX8-PPARgamma1 fusion oncogene in human thyroid carcinoma Science 2000;289:1357-60. [corrected]. [Crossref] [PubMed]
- Clinkscales W, Ong A, Nguyen S, et al. Diagnostic value of RAS mutations in indeterminate thyroid nodules. Otolaryngol Head Neck Surg 2017;156:472-9. [Crossref] [PubMed]
- Gupta N, Dasyam AK, Carty SE, et al. RAS mutations in thyroid FNA specimens are highly predictive of predominantly low-risk follicular-pattern cancers. J Clin Endocrinol Metab 2013;98:E914-22. [Crossref] [PubMed]
- Onenerk AM, Pusztaszeri MP, Canberk S, et al. Triage of the indeterminate thyroid aspirate: What are the options for the practicing cytopathologist? Cancer Cytopathol 2017;125:477-85. [PubMed]
- Bastos AU, Oler G, Nozima BHN, et al. BRAF V600E and decreased NIS and TPO expression are associated with aggressiveness of a subgroup of papillary thyroid microcarcinoma. Eur J Endocrinol 2015;173:525-40. [Crossref] [PubMed]
- Liu C, Chen T, Liu Z. Associations between BRAF(V600E) and prognostic factors and poor outcomes in papillary thyroid carcinoma: a meta-analysis. World J Surg Oncol 2016;14:241. [Crossref] [PubMed]
- Yip L, Nikiforova MN, Yoo JY, et al. Tumor genotype determines phenotype and disease-related outcomes in thyroid cancer: a study of 1510 patients. Ann Surg 2015;262:519-25; discussion 524-5. [Crossref] [PubMed]
- Kleiman DA, Sporn MJ, Beninato T, et al. Preoperative BRAF(V600E) mutation screening is unlikely to alter initial surgical treatment of patients with indeterminate thyroid nodules: a prospective case series of 960 patients. Cancer 2013;119:1495-502. [Crossref] [PubMed]
- Medici M, Kwong N, Angell TE, et al. The variable phenotype and low-risk nature of RAS-positive thyroid nodules. BMC Med 2015;13:184. [Crossref] [PubMed]
- Nikiforov YE. Molecular analysis of thyroid tumors. Mod Pathol 2011;24 Suppl 2:S34-43. [Crossref] [PubMed]
- Sugg SL, Zheng L, Rosen IB, et al. ret/PTC-1, -2, and -3 oncogene rearrangements in human thyroid carcinomas: implications for metastatic potential? J Clin Endocrinol Metab 1996;81:3360-5. [Crossref] [PubMed]
- Lam KY, Lo CY, Leung PS. High prevalence of RET proto-oncogene activation (RET/PTC) in papillary thyroid carcinomas. Eur J Endocrinol 2002;147:741-5. [Crossref] [PubMed]
- Romei C, Elisei R. RET/PTC Translocations and Clinico-Pathological Features in Human Papillary Thyroid Carcinoma. Front Endocrinol (Lausanne) 2012;3:54. [Crossref] [PubMed]
- Rodrigues HG, de Pontes AA, Adan LF. Use of molecular markers in samples obtained from preoperative aspiration of thyroid. Endocr J 2012;59:417-24. [Crossref] [PubMed]
- Nakazawa T, Kondo T, Kobayashi Y, et al. RET gene rearrangements (RET/PTC1 and RET/PTC3) in papillary thyroid carcinomas from an iodine-rich country (Japan). Cancer 2005;104:943-51. [Crossref] [PubMed]
- Nikiforov YE. RET/PTC rearrangement in thyroid tumors. Endocr Pathol 2002;13:3-16. [Crossref] [PubMed]
- Su X, Li Z, He C, et al. Radiation exposure, young age, and female gender are associated with high prevalence of RET/PTC1 and RET/PTC3 in papillary thyroid cancer: a meta-analysis. Oncotarget 2016;7:16716-30. [PubMed]
- Powell DJ, Russell J, Nibu K, et al. The RET/PTC3 oncogene: metastatic solid-type papillary carcinomas in murine thyroids. Cancer Res 1998;58:5523-8. [PubMed]
- Musholt TJ, Musholt PB, Khaladj N, et al. Prognostic significance of RET and NTRK1 rearrangements in sporadic papillary thyroid carcinoma. Surgery 2000;128:984-93. [Crossref] [PubMed]
- Basolo F, Molinaro E, Agate L, et al. RET protein expression has no prognostic impact on the long-term outcome of papillary thyroid carcinoma. Eur J Endocrinol 2001;145:599-604. [Crossref] [PubMed]
- Tallini G, Santoro M, Helie M, et al. RET/PTC oncogene activation defines a subset of papillary thyroid carcinomas lacking evidence of progression to poorly differentiated or undifferentiated tumor phenotypes. Clin Cancer Res 1998;4:287-94. [PubMed]
- French CA, Alexander EK, Cibas ES, et al. Genetic and biological subgroups of low-stage follicular thyroid cancer. Am J Pathol 2003;162:1053-60. [Crossref] [PubMed]
- Raman P, Koenig RJ. Pax-8-PPAR-γ fusion protein in thyroid carcinoma. Nat Rev Endocrinol 2014;10:616-23. [Crossref] [PubMed]
- Najafian A, Noureldine S, Azar F, et al. RAS Mutations, and RET/PTC and PAX8/PPAR-gamma Chromosomal Rearrangements Are Also Prevalent in Benign Thyroid Lesions: Implications Thereof and A Systematic Review. Thyroid 2017;27:39-48. [Crossref] [PubMed]
- Nikiforov YE, Carty SE, Chiosea SI, et al. Highly accurate diagnosis of cancer in thyroid nodules with follicular neoplasm/suspicious for a follicular neoplasm cytology by ThyroSeq v2 next-generation sequencing assay. Cancer 2014;120:3627-34. [Crossref] [PubMed]
- Labourier E, Shifrin A, Busseniers AE, et al. Molecular Testing for miRNA, mRNA, and DNA on Fine-Needle Aspiration Improves the Preoperative Diagnosis of Thyroid Nodules With Indeterminate Cytology. J Clin Endocrinol Metab 2015;100:2743-50. [Crossref] [PubMed]
- Ali SZ, Fish SA, Lanman R, et al. Use of the afirma® gene expression classifier for preoperative identification of benign thyroid nodules with indeterminate fine needle aspiration cytopathology. PLoS Curr 2013.5. [PubMed]
- Alexander EK, Kennedy GC, Baloch ZW, et al. Preoperative diagnosis of benign thyroid nodules with indeterminate cytology. N Engl J Med 2012;367:705-15. [Crossref] [PubMed]
- Nishino M. Molecular cytopathology for thyroid nodules: A review of methodology and test performance. Cancer Cytopathol 2016;124:14-27. [Crossref] [PubMed]
- Lithwick-Yanai G, Dromi N, Shtabsky A, et al. Multicentre validation of a microRNA-based assay for diagnosing indeterminate thyroid nodules utilising fine needle aspirate smears. J Clin Pathol 2017;70:500-7. [Crossref] [PubMed]
- Benjamin H, Schnitzer-Perlman T, Shtabsky A, et al. Analytical validity of a microRNA-based assay for diagnosing indeterminate thyroid FNA smears from routinely prepared cytology slides. Cancer Cytopathol 2016;124:711-21. [Crossref] [PubMed]
- Carthew RW, Sontheimer EJ. Origins and Mechanisms of miRNAs and siRNAs. Cell 2009;136:642-55. [Crossref] [PubMed]
- Pilli T, Cantara S, Marzocchi C, et al. Diagnostic Value of Circulating microRNA-95 and -190 in the Differential Diagnosis of Thyroid Nodules: A Validation Study in 1000 Consecutive Patients. Thyroid 2017;27:1053-7. [Crossref] [PubMed]
- Zhang Y, Xu D, Pan J, et al. Dynamic monitoring of circulating microRNAs as a predictive biomarker for the diagnosis and recurrence of papillary thyroid carcinoma. Oncol Lett 2017;13:4252-66. [Crossref] [PubMed]
- Hu J, Li C, Liu C, et al. Expressions of miRNAs in papillary thyroid carcinoma and their associations with the clinical characteristics of PTC. Cancer Biomark 2017;18:87-94. [Crossref] [PubMed]
- Keutgen XM, Filicori F, Crowley MJ, et al. A panel of four miRNAs accurately differentiates malignant from benign indeterminate thyroid lesions on fine needle aspiration. Clin Cancer Res 2012;18:2032-8. [Crossref] [PubMed]
- Cantara S, Marzocchi C, Pilli T, et al. Molecular signature of indeterminate thyroid lesions: current methods to improve fine needle aspiration cytology (FNAC) diagnosis. Int J Mol Sci 2017;18. [Crossref] [PubMed]
- Valderrabano P, Khazai L, Leon ME, et al. Evaluation of ThyroSeq v2 performance in thyroid nodules with indeterminate cytology. Endocr Relat Cancer 2017;24:127-36. [Crossref] [PubMed]
- Nikiforov YE, Carty SE, Chiosea SI, et al. Impact of the Multi-Gene ThyroSeq Next-Generation Sequencing Assay on Cancer Diagnosis in Thyroid Nodules with Atypia of Undetermined Significance/Follicular Lesion of Undetermined Significance Cytology. Thyroid 2015;25:1217-23. [Crossref] [PubMed]
- Randolph GW, Duh QY, Heller KS, et al. The prognostic significance of nodal metastases from papillary thyroid carcinoma can be stratified based on the size and number of metastatic lymph nodes, as well as the presence of extranodal extension. Thyroid 2012;22:1144-52. [Crossref] [PubMed]
- Choi JS, Kim J, Kwak JY, et al. Preoperative staging of papillary thyroid carcinoma: comparison of ultrasound imaging and CT. AJR Am J Roentgenol 2009;193:871-8. [Crossref] [PubMed]
- Ahn JE, Lee JH, Yi JS, et al. Diagnostic accuracy of CT and ultrasonography for evaluating metastatic cervical lymph nodes in patients with thyroid cancer. World J Surg 2008;32:1552-8. [Crossref] [PubMed]
- Lesnik D, Cunnane ME, Zurakowski D, et al. Papillary thyroid carcinoma nodal surgery directed by a preoperative radiographic map utilizing CT scan and ultrasound in all primary and reoperative patients. Head Neck 2014;36:191-202. [Crossref] [PubMed]
- Emerick GT, Duh QY, Siperstein AE, et al. Diagnosis, treatment, and outcome of follicular thyroid carcinoma. Cancer 1993;72:3287-95. [Crossref] [PubMed]
- D'Avanzo A, Treseler P, Ituarte PH, et al. Follicular thyroid carcinoma: histology and prognosis. Cancer 2004;100:1123-9. [Crossref] [PubMed]
- Bilimoria KY, Bentrem DJ, Ko CY, et al. Extent of surgery affects survival for papillary thyroid cancer. Ann Surg 2007;246:375-81; discussion 381-4. [Crossref] [PubMed]
- Grant CS, Hay ID, Gough IR, et al. Local recurrence in papillary thyroid carcinoma: is extent of surgical resection important? Surgery 1988;104:954-62. [PubMed]
- Mazzaferri EL, Kloos RT. Clinical review 128: Current approaches to primary therapy for papillary and follicular thyroid cancer. J Clin Endocrinol Metab 2001;86:1447-63. [Crossref] [PubMed]
- Adam MA, Pura J, Gu L, et al. Extent of surgery for papillary thyroid cancer is not associated with survival: an analysis of 61,775 patients. Ann Surg 2014;260:601-5; discussion 605-7. [Crossref] [PubMed]
- Matsuzu K, Sugino K, Masudo K, et al. Thyroid lobectomy for papillary thyroid cancer: long-term follow-up study of 1,088 cases. World J Surg 2014;38:68-79. [Crossref] [PubMed]
- Barney BM, Hitchcock YJ, Sharma P, et al. Overall and cause-specific survival for patients undergoing lobectomy, near-total, or total thyroidectomy for differentiated thyroid cancer. Head Neck 2011;33:645-9. [Crossref] [PubMed]
- Mendelsohn AH, Elashoff DA, Abemayor E, et al. Surgery for papillary thyroid carcinoma: is lobectomy enough? Arch Otolaryngol Head Neck Surg 2010;136:1055-61. [Crossref] [PubMed]
- Haigh PI, Urbach DR, Rotstein LE. Extent of thyroidectomy is not a major determinant of survival in low- or high-risk papillary thyroid cancer. Ann Surg Oncol 2005;12:81-9. [Crossref] [PubMed]
- Vaisman F, Shaha A, Fish S, et al. Initial therapy with either thyroid lobectomy or total thyroidectomy without radioactive iodine remnant ablation is associated with very low rates of structural disease recurrence in properly selected patients with differentiated thyroid cancer. Clin Endocrinol (Oxf) 2011;75:112-9. [Crossref] [PubMed]
- Kluijfhout WP, Pasternak JD, Drake FT, et al. Application of the new American Thyroid Association guidelines leads to a substantial rate of completion total thyroidectomy to enable adjuvant radioactive iodine. Surgery 2017;161:127-33. [Crossref] [PubMed]
- Pasieka JL, Thompson NW, McLeod MK, et al. The incidence of bilateral well-differentiated thyroid cancer found at completion thyroidectomy. World J Surg 1992;16:711-6; discussion 716-7. [Crossref] [PubMed]
- Mazeh H, Samet Y, Hochstein D, et al. Multifocality in well-differentiated thyroid carcinomas calls for total thyroidectomy. Am J Surg 2011;201:770-5. [Crossref] [PubMed]
- Kim BY, Choi JE, Lee E, et al. Prognostic factors for recurrence of locally advanced differentiated thyroid cancer. J Surg Oncol 2017;116:877-83.
- Wang W, Su X, He K, et al. Comparison of the clinicopathologic features and prognosis of bilateral versus unilateral multifocal papillary thyroid cancer: An updated study with more than 2000 consecutive patients. Cancer 2016;122:198-206. [Crossref] [PubMed]
- Al Afif A, Williams BA, Rigby MH, et al. Multifocal papillary thyroid cancer increases the risk of central lymph node metastasis. Thyroid 2015;25:1008-12. [Crossref] [PubMed]
- Kim KJ, Kim SM, Lee YS, et al. Prognostic significance of tumor multifocality in papillary thyroid carcinoma and its relationship with primary tumor size: a retrospective study of 2,309 consecutive patients. Ann Surg Oncol 2015;22:125-31. [Crossref] [PubMed]
- Jonklaas J. Role of radioactive iodine for adjuvant therapy and treatment of metastases. J Natl Compr Canc Netw 2007;5:631-40. [Crossref] [PubMed]
- Pelikan DM, Lion HL, Hermans J, et al. The role of radioactive iodine in the treatment of advanced differentiated thyroid carcinoma. Clin Endocrinol (Oxf) 1997;47:713-20. [Crossref] [PubMed]
- Samaan NA, Schultz PN, Haynie TP, et al. Pulmonary metastasis of differentiated thyroid carcinoma: treatment results in 101 patients. J Clin Endocrinol Metab 1985;60:376-80. [Crossref] [PubMed]
- Seidlin SM, Marinelli LD, Oshry E. Radioactive iodine therapy; effect on functioning metastases of adenocarcinoma of the thyroid. J Am Med Assoc 1946;132:838-47. [Crossref] [PubMed]
- Clement SC, Peeters RP, Ronckers CM, et al. Intermediate and long-term adverse effects of radioiodine therapy for differentiated thyroid carcinoma--a systematic review. Cancer Treat Rev 2015;41:925-34. [Crossref] [PubMed]
- Florenzano P, Guarda FJ, Jaimovich R, et al. Radioactive Iodine Administration Is Associated with Persistent Related Symptoms in Patients with Differentiated Thyroid Cancer. Int J Endocrinol 2016;2016. [Crossref] [PubMed]
- Fard-Esfahani A, Emami-Ardekani A, Fallahi B, et al. Adverse effects of radioactive iodine-131 treatment for differentiated thyroid carcinoma. Nucl Med Commun 2014;35:808-17. [PubMed]
- Mitchell AL, Gandhi A, Scott-Coombes D, et al. Management of thyroid cancer: United Kingdom National Multidisciplinary Guidelines. J Laryngol Otol 2016;130:S150-60. [Crossref] [PubMed]
- Silberstein EB, Alavi A, Balon HR, et al. The SNMMI practice guideline for therapy of thyroid disease with 131I 3.0. J Nucl Med 2012;53:1633-51. [Crossref] [PubMed]
- Ruel E, Thomas S, Dinan M, et al. Adjuvant radioactive iodine therapy is associated with improved survival for patients with intermediate-risk papillary thyroid cancer. J Clin Endocrinol Metab 2015;100:1529-36. [Crossref] [PubMed]
- Kazaure HS, Roman SA, Sosa JA. Insular thyroid cancer: a population-level analysis of patient characteristics and predictors of survival. Cancer 2012;118:3260-7. [Crossref] [PubMed]
- Kazaure HS, Roman SA, Sosa JA. Aggressive variants of papillary thyroid cancer: incidence, characteristics and predictors of survival among 43,738 patients. Ann Surg Oncol 2012;19:1874-80. [Crossref] [PubMed]
- Zhang H, Cai Y, Zheng L, et al. Postoperative radioactive iodine-131 ablation is not necessary among patients with intermediate-risk differentiated thyroid carcinoma: a population-based study. Hell J Nucl Med 2017;20:3-10. [PubMed]
- Kim SK, Woo JW, Lee JH, et al. Radioactive iodine ablation may not decrease the risk of recurrence in intermediate-risk papillary thyroid carcinoma. Endocr Relat Cancer 2016;23:367-76. [Crossref] [PubMed]
- Mallick U, Harmer C, Yap B, et al. Ablation with low-dose radioiodine and thyrotropin alfa in thyroid cancer. N Engl J Med 2012;366:1674-85. [Crossref] [PubMed]
- Schlumberger M, Catargi B, Borget I, et al. Strategies of radioiodine ablation in patients with low-risk thyroid cancer. N Engl J Med 2012;366:1663-73. [Crossref] [PubMed]
- Mäenpää HO, Heikkonen J, Vaalavirta L, et al. Low vs. high radioiodine activity to ablate the thyroid after thyroidectomy for cancer: a randomized study. PLoS One 2008;3. [Crossref] [PubMed]
- Fallahi B, Beiki D, Takavar A, et al. Low versus high radioiodine dose in postoperative ablation of residual thyroid tissue in patients with differentiated thyroid carcinoma: a large randomized clinical trial. Nucl Med Commun 2012;33:275-82. [Crossref] [PubMed]
- Busaidy NL, Cabanillas ME. Differentiated thyroid cancer: management of patients with radioiodine nonresponsive disease. J Thyroid Res 2012;2012. [Crossref] [PubMed]
- Haugen BR. Management of the patient with progressive radioiodine non-responsive disease. Semin Surg Oncol 1999;16:34-41. [Crossref] [PubMed]
- Droz JP, Schlumberger M, Rougier P, et al. Chemotherapy in metastatic nonanaplastic thyroid cancer: experience at the Institut Gustave-Roussy. Tumori 1990;76:480-3. [Crossref] [PubMed]
- Jasim S, Ozsari L, Habra MA. Multikinase inhibitors use in differentiated thyroid carcinoma. Biologics 2014;8:281-91. [PubMed]
- Narayanan S, Colevas AD. Current standards in treatment of radioiodine refractory thyroid cancer. Curr Treat Options Oncol 2016;17:30. [Crossref] [PubMed]
- Lorusso L, Pieruzzi L, Biagini A, et al. Lenvatinib and other tyrosine kinase inhibitors for the treatment of radioiodine refractory, advanced, and progressive thyroid cancer. Onco Targets Ther 2016;9:6467-77. [Crossref] [PubMed]
- Fallahi P, Ferrari SM, Di Bari F, et al. Cabozantinib in thyroid cancer. Recent Pat Anticancer Drug Discov 2015;10:259-69. [Crossref] [PubMed]
- Fallahi P, Di Bari F, Ferrari SM, et al. Selective use of vandetanib in the treatment of thyroid cancer. Drug Des Devel Ther 2015;9:3459-70. [PubMed]
- Hewett Y, Ghimire S, Farooqi B, et al. Lenvatinib - A multikinase inhibitor for radioiodine-refractory differentiated thyroid cancer. J Oncol Pharm Pract 2018;24:28-32. [Crossref] [PubMed]
- Corrado A, Ferrari SM, Politti U, et al. Aggressive thyroid cancer: targeted therapy with sorafenib. Minerva Endocrinol 2017;42:64-76. [PubMed]
- Frasca F, Vella V, Nicolosi ML, et al. Thyroid cancer cell resistance to gefitinib depends on the constitutive oncogenic activation of the ERK pathway. J Clin Endocrinol Metab 2013;98:2502-12. [Crossref] [PubMed]
- Johnson GL, Stuhlmiller TJ, Angus SP, et al. Molecular pathways: adaptive kinome reprogramming in response to targeted inhibition of the BRAF-MEK-ERK pathway in cancer. Clin Cancer Res 2014;20:2516-22. [Crossref] [PubMed]
- Dadu R, Devine C, Hernandez M, et al. Role of salvage targeted therapy in differentiated thyroid cancer patients who failed first-line sorafenib. J Clin Endocrinol Metab 2014;99:2086-94. [Crossref] [PubMed]
- Chrisoulidou A, Mandanas S, Margaritidou E, et al. Treatment compliance and severe adverse events limit the use of tyrosine kinase inhibitors in refractory thyroid cancer. Onco Targets Ther 2015;8:2435-42. [PubMed]
- Frampton JE. Lenvatinib: A review in refractory thyroid cancer. Target Oncol 2016;11:115-22. [Crossref] [PubMed]
- Schlumberger M, Tahara M, Wirth LJ, et al. Lenvatinib versus placebo in radioiodine-refractory thyroid cancer. N Engl J Med 2015;372:621-30. [Crossref] [PubMed]
- Patel AB, O’Hare T, Deininger MW. Mechanisms of Resistance to ABL Kinase Inhibition in Chronic Myeloid Leukemia and the Development of Next Generation ABL Kinase Inhibitors. Hematol Oncol Clin North Am 2017;31:589-612. [Crossref] [PubMed]
- Liao BC, Lin CC, Lee JH, et al. Optimal management of EGFR-mutant non-small cell lung cancer with disease progression on first-line tyrosine kinase inhibitor therapy. Lung Cancer 2017;110:7-13. [Crossref] [PubMed]
- Yao TW, Zhang J, Prados M, et al. Acquired resistance to BRAF inhibition in BRAFV600E mutant gliomas. Oncotarget 2017;8:583-95. [PubMed]
- Beadnell TC, Mishall KM, Zhou Q, et al. The Mitogen-Activated Protein Kinase Pathway Facilitates Resistance to the Src Inhibitor Dasatinib in Thyroid Cancer. Mol Cancer Ther 2016;15:1952-63. [Crossref] [PubMed]
- Gao WL, Wie LL, Chao YG, et al. Prognostic prediction of BRAF(V600E) and its relationship with sodium iodide symporter in classic variant of papillary thyroid carcinomas. Clin Lab 2012;58:919-26. [PubMed]
- Kleiman DA, Buitrago D, Crowley MJ, et al. Thyroid stimulating hormone increases iodine uptake by thyroid cancer cells during BRAF silencing. J Surg Res 2013;182:85-93. [Crossref] [PubMed]
- Ho AL, Grewal RK, Leboeuf R, et al. Selumetinib-enhanced radioiodine uptake in advanced thyroid cancer. N Engl J Med 2013;368:623-32. [Crossref] [PubMed]
- Yang K, Wang H, Liang Z, et al. BRAFV600E mutation associated with non-radioiodine-avid status in distant metastatic papillary thyroid carcinoma. Clin Nucl Med 2014;39:675-9. [Crossref] [PubMed]
- Chakravarty D, Santos E, Ryder M, et al. Small-molecule MAPK inhibitors restore radioiodine incorporation in mouse thyroid cancers with conditional BRAF activation. J Clin Invest 2011;121:4700-11. [Crossref] [PubMed]
- Lakshmanan A, Scarberry D, Green JA, et al. Modulation of thyroidal radioiodide uptake by oncological pipeline inhibitors and Apigenin. Oncotarget 2015;6:31792-804. [Crossref] [PubMed]
- Haugen BR, Sawka AM, Alexander EK, et al. American Thyroid Association Guidelines on the Management of Thyroid Nodules and Differentiated Thyroid Cancer Task Force Review and Recommendation on the Proposed Renaming of Encapsulated Follicular Variant Papillary Thyroid Carcinoma Without Invasion to Noninvasive Follicular Thyroid Neoplasm with Papillary-Like Nuclear Features. Thyroid 2017;27:481-3. [Crossref] [PubMed]