A reappraisal of vascular anatomy of the parathyroid gland based on fluorescence techniques
Vascular anatomy of the parathyroid glands (PGs) and its importance in neck endocrine surgery
Knowing the anatomical position and vascular supply of the PGs is essential to avoid hypoparathyroidism after thyroid surgery (1,2). Temporary hypoparathyroidism with resulting hypocalcemia is the most common complication after total thyroidectomy and occurs in up to 30% of patients who undergo total thyroidectomy (3,4). Its incidence depends on the technical difficulty of the procedure and expertise of the surgeon. Permanent hypocalcemia, defined as hypocalcemia for more than 6 months after thyroidectomy, is reported in 1–10% of patients (5,6). Reducing the rate of hypoparathyroidism is essential for improving the quality of life, as postoperative hypocalcemia can result in prolonged hospitalization and multiple clinic visits, neuromuscular symptoms, the need for life-long calcium and vitamin D supplementation, and long-term complications, such as cerebral, vascular, ocular, and renal damage (7-11).
In a study of 100 cadaveric thyroid glands by Delattre et al. (12), 38.2% of the parathyroid feeding vessels were considered at risk for damage by dissection during standard thyroidectomy. Furthermore, all four PGs were at risk in 5% of the cadavers, with the superior glands at increased risk, as they usually presented with a shorter feeding vessel and were tightly positioned at the posterior side of the upper pole of the thyroid. Knowledge about the origin and course of arterial supply is thus of utmost importance. The authors found a single feeding vessel to the PGs in 80% of cases. In general, both the superior and inferior PGs received their blood supply from the inferior thyroid artery (ITA): the superior PGs received blood supply from the ITA in 77% of cases, from the superior thyroid artery (STA) in 15%, and from anastomoses between the two arteries, running posteriorly to the thyroid in 8%. The inferior PGs were supplied by the ITA in 90.3% of cases, and by the STA in 5%. The authors found an absent ITA in 4.5% of cases; in such cases an anterior running vessel from the STA is particularly at risk during lobectomy (Figure 1A,B). These findings highlight the importance of considering the vascular supply as an interconnected network (loop of anastomoses) of vessels, possibly running close to the thyroid parenchyma and often with short branches connecting to or traversing the thyroid parenchyma. Such cases are at risk for devascularisation, even when a long feeding pedicle is present (Figure 2A,B).
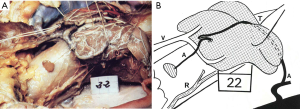
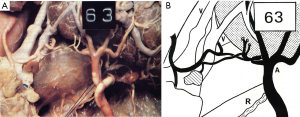
Furthermore, Delattre et al. (12) reported that they were able to locate a greater number of PGs (at least all 4 PGs) during their last 40 cases of micro-dissection, suggesting that the experience of the surgeon might play an important role in PG localization and preservation. In addition, the authors concluded that the position of the parathyroid artery in relation to the thyroid parenchyma is the most important factor when considering the risk of devascularisation during lobectomy, rather than the length of the artery. Since these arteries are terminal vessels, systematic identification, precise surgical dissection, and micro-ligatures are key in reducing the frequency of iatrogenic hypoparathyroidism (12), a risk that was first described by Halsted et al. in 1907 (13). These results provide an anatomic explanation for the consistent 1–10% of definitive hypoparathyroidism reported in most registry-based or multicenter studies (5,14).
Hypocalcemia after total thyroidectomy might result from intraoperative damage to the PGs due to trauma, inadvertent removal, or devascularisation. The extent of damage to the PGs is difficult to predict intraoperatively. It is generally accepted that sufficient parathyroid hormone (PTH) can be produced by one half of a normal PG (6). To avoid postoperative hypocalcemia, parathyroid auto-transplantation can be performed; however, the results have been inconsistent, creating controversy (15-17). Therefore, capsular dissection techniques and preservation of the surrounding vasculature have been proposed and utilized, in an attempt to avoid inadvertent parathyroidectomy or the disruption of the parathyroid vasculature (18-20). Several studies have shown a reduction in transient and permanent hypoparathyroidism after adopting vascular-preservation techniques and classification systems that guide dissection, resection, and decisions regarding auto-transplantation (19,21).
Preservation of the PGs can be challenging, as normal postoperative parathyroid function is not guaranteed even when the PGs are thought to be well preserved during surgery. A study by Lang et al. (22) examined 103 patients who underwent total thyroidectomy with identification of all 4 PGs and a visual analysis of the PGs. The authors reported that having more than 3 discolored PGs was an independent risk factor for transient hypoparathyroidism. However, 12.5% of patients with 4 normally colored PGs, assumed to be fully functioning, presented with hypoparathyroidism. The authors concluded that PG discoloration is associated with transient hypoparathyroidism, and that normally colored PGs with assumed adequate blood supply do not necessarily imply a functional gland (22). The authors also highlighted the need for individual real-time intraoperative methods to assess PG viability.
Angiography with indocyanine green (ICG) can be used as adjunct technique to help identify the vascular blood supply of the PGs at risk for damage during thyroid gland dissection and to aid in the prediction of the functionality of the identified PGs.
Fluorescent techniques with ICG in neck surgery
Accurate prediction of post-thyroidectomy hypocalcemia might lead to a modification of surgical strategies. However, there is a need for reliable tools that can accurately predict whether a patient will develop hypocalcemia (5,23,24). The current techniques for evaluating parathyroid function are based on calcium (25,26) and PTH (6,27-31) measurements at various time points during or after thyroidectomy. Some studies have suggested that early (a few minutes to 12 hours after thyroid resection) PTH measurements reliably predict the absence of hypoparathyroidism, with a positive predictive value up to 97% (6,27,28). However, this finding has been challenged by other authors (32,33). Unlike ICG-angiography, which has immediate results, calcium and PTH level measurements are usually not able to guide intra-operative decision-making, as their results require a long time to develop. However, some authors have suggested using quick PTH measurements to demonstrate parathyroid insufficiency, providing results that can then help surgeons decide whether to auto-transplant a PG (29,34).
ICG is a water-soluble, 775 Da-sized molecule with a maximum absorption spectrum of 805 nm and re-emission at 835 nm when excited by a light/laser at a wavelength in the near-infrared (NIR) spectrum. Once injected, ICG becomes completely and permanently fixed to plasmatic proteins in the bloodstream, and circulates in the intravascular compartment only. It has a half-life of 3.4±0.7 minutes, and is taken up from the plasma almost exclusively by the hepatic parenchymal cells, before being secreted entirely into the bile. Iodine allergy is a contra-indication for ICG administration, as iodine is present in its molecular structure. The largest study performed to date found that allergic reactions occur in 1/80,000 patients who receive ICG (35).
Initially ICG was used in ophthalmology for the detection of macular degeneration (36). Subsequently, ICG angiography has been used to identify sentinel lymph nodes (37), determine the extent of oncologic resections (38), and study hepatic function (39). Recent studies have also demonstrated its usefulness in evaluating the vascular blood flow of intestinal anastomoses (40) and tissue flap reconstructions (41).
At our center, ICG for thyroid or parathyroid surgery is prepared according to the protocols used for abdominal surgery (38). Briefly, 25 mg ICG is mixed with 10 mL of sterile water (concentration, 2.5 mg/mL), and 3.5 mL is injected intravenously during the procedure by the anesthesia team. The injection can be repeated until a maximum dose of 5 mg/kg per day is reached. The catheter is then purged after each injection for rapid imaging gain. After approximately 1–2 minutes, images are acquired using a laparoscopic NIR PinPoint® camera (Novadaq, Ontario, Canada).
One benefit of the use of ICG technology is that the anatomy of the feeding vessels to the PGs can be analyzed prior to performing a thyroid lobectomy, allowing the preservation of the vessel loops feeding the PGs (Figures 3,4). The video shows a case of thyroidectomy for malignant disease. After ICG angiography and the visualization of the feeding vessel loop attached to the thyroid, a precise capsular dissection technique was performed and a tiny thyroid remnant was left behind in order not to harm the vessel loop and thus preserve the PG.
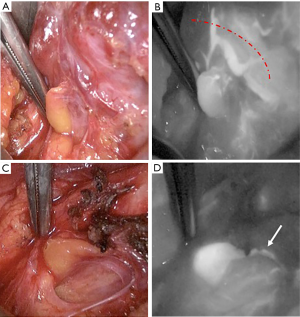
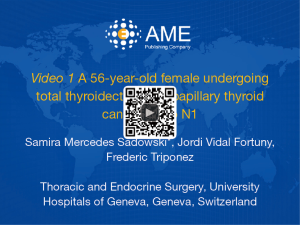
We have systematically utilized standardized ICG angiography in hundreds of cases and are able to perform a vascular mapping of the PG feeding vessels. This has made us more clearly aware of the anatomy and locations of the PGs, as well as the presence of vascular loops, which are often very close to the thyroid parenchyma (Figures 1,2). Thus, we currently perform dissections for PG preservation in a very precise and arduous manner in terms of capsular dissection, occasionally leaving behind a small thyroid remnant to preserve the attached PG vessel loops. Whether this technique will further reduce postoperative hypoparathyroidism needs to be evaluated in future studies.
Application of ICG in thyroid and parathyroid surgery for the evaluation of PG function
The PGs need to be identified early during the thyroidectomy dissection, and their vascular supply must be preserved to prevent postoperative hypocalcemia. The use of ICG for PG identification during thyroid surgery was first proposed in a study by Suh et al. (43) in 2014, in which the authors showed that the PGs could be visualized using ICG NIR imaging in dogs. In the same year, another group (44) managed to differentially visualize the thyroid and PGs using NIR imaging in pigs.
In our initial experience using ICG to evaluate intraoperative perfusion of the PGs for the prediction of parathyroid function after thyroidectomy (45), we demonstrated that the presence of one well-perfused PG or a well-perfused PG remnant was sufficient to avoid hypoparathyroidism (46). After total thyroidectomy, we found that at least one well-perfused gland was present on angiography in 30 of 36 patients; none of the 30 patients experienced postoperative hypoparathyroidism. On the other hand, transient postoperative hypoparathyroidism was noted in two of the six patients who did not have at least one well-perfused PG on angiography. In cases of discrepancy between the visual assessment and ICG angiography, an incision was made on the PG and glands that did not bleed were auto-transplanted (five cases).
In addition, we have shown the superiority of ICG angiography over visual assessment. In our preliminary study, 71 of 101 PGs were visually evaluated as well-vascularized, while only 51 were considered well-vascularized on ICG angiography (45). Thus, the perfusion status (and therefore the functional ability to produce PTH) was visually over-evaluated in 20 of 71 PGs (28.2%). Similar findings have been reported for 27 patients who underwent thyroidectomy (47). In this prospective study, a total of 84% visually identified PGs showed ICG uptake. PG perfusion was scored both visually and by ICG fluorescence. A discrepancy between visual and ICG scores was noted in 6% of cases. In addition, three patients had transient postoperative hypocalcemia, with only one patient being symptomatic. It should be noted that the utility of ICG is limited in patients with a present thyroid gland, as the parathyroid fluorescence is frequently obscured by the thyroid.
In 2017, Lang et al. (48) studied postoperative hypocalcemia after total thyroidectomy and its correlation with fluorescence intensity on ICG angiography, using the SPY® Fluorescent Imaging System (Novadaq Technologies, Inc.). The authors evaluated a total of 324 biopsy-confirmed PGs from 94 patients. The fluorescence intensity of each PG was expressed as the fluorescence intensity ratio between the PG and the anterior trachea, and the greatest fluorescence intensity (GFI) was assessed. The GFI value was found to be the best predictor of early postoperative hypocalcemia (0% chance of hypocalcemia for a GFI value >150% vs. 81.8% chance of hypocalcemia for a GFI value ≤150%). There were no cases of permanent hypocalcemia, regardless of GFI value (48).
One of the limitations of many studies analyzing ICG angiography during thyroidectomy is the fact that in most patients, not all 4 PGs are evaluated. Therefore, in those patients with less than 4 evaluated PGs, the perfusion and function of the non-visualized PGs remain unknown, obscuring a clear correlation between ICG perfusion (evaluated in 1, 2 or 3 PGs) and postoperative PTH levels (reflecting the function of all 4 PGs). We therefore analyzed patients undergoing subtotal parathyroidectomy (49) and reported our findings on ICG use in a prospective study of 13 patients undergoing subtotal parathyroidectomy for multiglandular disease (primary and secondary hyperparathyroidism) (46). Our goal was to determine whether postoperative single PG (or PG remnant) function was truly reflected by intraoperative ICG angiography. For this purpose, only cases with all four PGs visualized were included. The PG that was to be preserved was selected based on the degree of perfusion on ICG angiography. When the gland chosen by the surgeon visually showed poor perfusion on angiography, another gland was selected for preservation. On follow-up, normal levels of PTH were achieved in all patients, demonstrating that the well-perfused PG or remnant was functional.
In 2016, Zaidi et al. (50) published results of a prospective study involving 33 patients who underwent surgery for primary hyperparathyroidism. This study included both parathyroid adenoma excisions as well as subtotal parathyroidectomies (3.5-gland excision). Overall, 92.9% of the identified PGs visually demonstrated ICG uptake. In most cases, the presence of thyroid tissue limited the parathyroid fluorescence, as it has high vascular ICG uptake. The authors found ICG angiography to be useful in remnant PG function assessment in cases of subtotal parathyroidectomies and in patients who had a previous thyroidectomy.
Future directions, proposals and conclusions
The largely encouraging findings in the above studies, led us to design a prospective, randomized study to determine whether the systematic measurement of calcium and PTH levels, as well as the systematic supplementation of calcium and vitamin D therapy, can be omitted in patients with at least one well-perfused PG identified on ICG angiography after the thyroid gland has been removed. We hypothesized that patients with a well-perfused PG, as demonstrated via ICG angiography, would not develop postoperative hypoparathyroidism and therefore would not need post-operative calcium and/or PTH measurements nor calcium and vitamin D supplementation. The results of this study should be available soon.
There are several areas in this field that require further development. The technique could be further improved, especially regarding standardization, which would allow for universal applications and a more objective scoring system. In addition, cost-benefit analyses need to be performed. The material is costly; however, the cost can be shared by all departments (abdominal, gynecology, plastic and neck surgery), as in our institution. Fortunately, the materials can even be shared in high volume centers, as the ICG procedure itself takes less than 5 minutes.
In conclusion, we believe that the use of ICG angiography of the PGs during thyroid surgery can lead to a reduction in the rate of postoperative hypoparathyroidism. First, ICG angiography allows surgeons to adapt their technique for PG preservation depending on parathyroid perfusion and vascular anatomy. Second, ICG angiography allows surgeons to verify the perfusion of the PGs after thyroid resection, indicating whether a PG should be auto-transplanted. ICG angiography is currently the only available real-time tool able to intraoperatively predict the function of each individual PG, and can therefore assist surgeons in their decision-making regarding how to avoid post-thyroidectomy hypoparathyroidism.
Acknowledgements
We thank Mark Licker, Christoph Ellenberger and John Diaper from the Anesthesiology Department for their support.
Footnote
Conflicts of Interest: F Triponez received travel grants from Novadaq. The other authors have no conflicts of interest to declare.
References
- Wang C. The anatomic basis of parathyroid surgery. Ann Surg 1976;183:271-5. [Crossref] [PubMed]
- Nobori M, Saiki S, Tanaka N, et al. Blood supply of the parathyroid gland from the superior thyroid artery. Surgery 1994;115:417-23. [PubMed]
- Puzziello A, Rosato L, Innaro N, et al. Hypocalcemia following thyroid surgery: incidence and risk factors. A longitudinal multicenter study comprising 2,631 patients. Endocrine 2014;47:537-42. [Crossref] [PubMed]
- Zambudio AR, Rodriguez J, Riquelme J, et al. Prospective study of postoperative complications after total thyroidectomy for multinodular goiters by surgeons with experience in endocrine surgery. Ann Surg 2004;240:18-25. [Crossref] [PubMed]
- Edafe O, Antakia R, Laskar N, et al. Systematic review and meta-analysis of predictors of post-thyroidectomy hypocalcaemia. Br J Surg 2014;101:307-20. [Crossref] [PubMed]
- Lorente-Poch L, Sancho JJ, Munoz-Nova JL, et al. Defining the syndromes of parathyroid failure after total thyroidectomy. Gland Surg 2015;4:82-90. [PubMed]
- Thomusch O, Machens A, Sekulla C, et al. The impact of surgical technique on postoperative hypoparathyroidism in bilateral thyroid surgery: a multivariate analysis of 5846 consecutive patients. Surgery 2003;133:180-5. [Crossref] [PubMed]
- Shoback D. Clinical practice. Hypoparathyroidism. N Engl J Med 2008;359:391-403. [Crossref] [PubMed]
- Zarnegar R, Brunaud L, Clark OH. Prevention, evaluation, and management of complications following thyroidectomy for thyroid carcinoma. Endocrinol Metab Clin North Am 2003;32:483-502. [Crossref] [PubMed]
- Arlt W, Fremerey C, Callies F, et al. Well-being, mood and calcium homeostasis in patients with hypoparathyroidism receiving standard treatment with calcium and vitamin D. Eur J Endocrinol 2002;146:215-22. [Crossref] [PubMed]
- Underbjerg L, Sikjaer T, Mosekilde L, et al. Cardiovascular and renal complications to postsurgical hypoparathyroidism: a Danish nationwide controlled historic follow-up study. J Bone Miner Res 2013;28:2277-85. [Crossref] [PubMed]
- Delattre JF, Flament JB, Palot JP, et al. Variations in the parathyroid glands. Number, situation and arterial vascularization. Anatomical study and surgical application. J Chir (Paris) 1982;119:633-41. [PubMed]
- Halsted WS, Evans HM. I. The Parathyroid Glandules. Their Blood Supply and their Preservation in Operation upon the Thyroid Gland. Ann Surg 1907;46:489-506. [Crossref] [PubMed]
- Duclos A, Peix JL, Colin C, et al. Influence of experience on performance of individual surgeons in thyroid surgery: prospective cross sectional multicentre study. BMJ 2012;344:d8041. [Crossref] [PubMed]
- Lorente-Poch L, Sancho JJ, Ruiz S, et al. Importance of in situ preservation of parathyroid glands during total thyroidectomy. Br J Surg 2015;102:359-67. [Crossref] [PubMed]
- Olson JA Jr, DeBenedetti MK, Baumann DS, et al. Parathyroid autotransplantation during thyroidectomy. Results of long-term follow-up. Ann Surg 1996;223:472-8; discussion 478-80. [Crossref] [PubMed]
- Promberger R, Ott J, Kober F, et al. Intra- and postoperative parathyroid hormone-kinetics do not advocate for autotransplantation of discolored parathyroid glands during thyroidectomy. Thyroid 2010;20:1371-5. [Crossref] [PubMed]
- Bliss RD, Gauger PG, Delbridge LW. Surgeon's approach to the thyroid gland: surgical anatomy and the importance of technique. World J Surg 2000;24:891-7. [Crossref] [PubMed]
- Park I, Rhu J, Woo JW, et al. Preserving Parathyroid Gland Vasculature to Reduce Post-thyroidectomy Hypocalcemia. World J Surg 2016;40:1382-9. [Crossref] [PubMed]
- Delbridge L, Reeve TS, Khadra M, et al. Total thyroidectomy: the technique of capsular dissection. Aust N Z J Surg 1992;62:96-9. [Crossref] [PubMed]
- Cui Q, Li Z, Kong D, et al. A prospective cohort study of novel functional types of parathyroid glands in thyroidectomy: In situ preservation or auto-transplantation? Medicine (Baltimore) 2016;95:e5810. [Crossref] [PubMed]
- Lang BH, Chan DT, Chow FC, et al. The Association of Discolored Parathyroid Glands and Hypoparathyroidism Following Total Thyroidectomy. World J Surg 2016;40:1611-7. [Crossref] [PubMed]
- Almquist M, Hallgrimsson P, Nordenstrom E, et al. Prediction of permanent hypoparathyroidism after total thyroidectomy. World J Surg 2014;38:2613-20. [Crossref] [PubMed]
- Julián MT, Balibrea JM, Granada ML, et al. Intact parathyroid hormone measurement at 24 hours after thyroid surgery as predictor of parathyroid function at long term. Am J Surg 2013;206:783-9. [Crossref] [PubMed]
- Adams J, Andersen P, Everts E, et al. Early postoperative calcium levels as predictors of hypocalcemia. Laryngoscope 1998;108:1829-31. [Crossref] [PubMed]
- Marohn MR, LaCivita KA. Evaluation of total/near-total thyroidectomy in a short-stay hospitalization: safe and cost-effective. Surgery 1995;118:943-7; discussion 947-8. [Crossref] [PubMed]
- Terris DJ, Snyder S, Carneiro-Pla D, et al. American Thyroid Association statement on outpatient thyroidectomy. Thyroid 2013;23:1193-202. [Crossref] [PubMed]
- Cmilansky P, Mrozova L. Hypocalcemia - the most common complication after total thyroidectomy. Bratisl Lek Listy 2014;115:175-8. [PubMed]
- Lo CY, Luk JM, Tam SC. Applicability of intraoperative parathyroid hormone assay during thyroidectomy. Ann Surg 2002;236:564-9. [Crossref] [PubMed]
- Lang BH, Yih PC, Ng KK. A prospective evaluation of quick intraoperative parathyroid hormone assay at the time of skin closure in predicting clinically relevant hypocalcemia after thyroidectomy. World J Surg 2012;36:1300-6. [Crossref] [PubMed]
- Gupta S, Chaudhary P, Durga CK, et al. Validation of intra-operative parathyroid hormone and its decline as early predictors of hypoparathyroidism after total thyroidectomy: A prospective cohort study. Int J Surg 2015;18:150-3. [Crossref] [PubMed]
- Hermann M, Ott J, Promberger R, et al. Kinetics of serum parathyroid hormone during and after thyroid surgery. Br J Surg 2008;95:1480-7. [Crossref] [PubMed]
- Lombardi CP, Raffaelli M, Princi P, et al. Parathyroid hormone levels 4 hours after surgery do not accurately predict post-thyroidectomy hypocalcemia. Surgery 2006;140:1016-23; discussion 1023-5. [Crossref] [PubMed]
- Barczyński M, Cichon S, Konturek A, et al. Applicability of intraoperative parathyroid hormone assay during total thyroidectomy as a guide for the surgeon to selective parathyroid tissue autotransplantation. World J Surg 2008;32:822-8. [Crossref] [PubMed]
- Hope-Ross M, Yannuzzi LA, Gragoudas ES, et al. Adverse reactions due to indocyanine green. Ophthalmology 1994;101:529-33. [Crossref] [PubMed]
- de Boer E, Harlaar NJ, Taruttis A, et al. Optical innovations in surgery. Br J Surg 2015;102:e56-72. [Crossref] [PubMed]
- Imboden S, Papadia A, Nauwerk M, et al. A Comparison of Radiocolloid and Indocyanine Green Fluorescence Imaging, Sentinel Lymph Node Mapping in Patients with Cervical Cancer Undergoing Laparoscopic Surgery. Ann Surg Oncol 2015;22:4198-203. [Crossref] [PubMed]
- Ishizawa T, Fukushima N, Shibahara J, et al. Real-time identification of liver cancers by using indocyanine green fluorescent imaging. Cancer 2009;115:2491-504. [Crossref] [PubMed]
- Halle BM, Poulsen TD, Pedersen HP. Indocyanine green plasma disappearance rate as dynamic liver function test in critically ill patients. Acta Anaesthesiol Scand 2014;58:1214-9. [Crossref] [PubMed]
- Ris F, Hompes R, Lindsey I, et al. Near infra-red laparoscopic assessment of the adequacy of blood perfusion of intestinal anastomosis - a video vignette. Colorectal Dis 2014;16:646-7. [Crossref] [PubMed]
- Eguchi T, Kawaguchi K, Basugi A, et al. Intraoperative real-time assessment of blood flow using indocyanine green angiography after anastomoses in free-flap reconstructions. Br J Oral Maxillofac Surg 2017;55:628-30. [Crossref] [PubMed]
- Sadowski SM, Fortuny JV, Triponez F. A 56-year-old female undergoing total thyroidectomy for papillary thyroid cancer, pT1b N1. Asvide 2017;4:533. Available online: http://www.asvide.com/articles/1853
- Suh YJ, Choi JY, Chai YJ, et al. Indocyanine green as a near-infrared fluorescent agent for identifying parathyroid glands during thyroid surgery in dogs. Surg Endosc 2015;29:2811-7. [Crossref] [PubMed]
- Hyun H, Park MH, Owens EA, et al. Structure-inherent targeting of near-infrared fluorophores for parathyroid and thyroid gland imaging. Nat Med 2015;21:192-7. [Crossref] [PubMed]
- Vidal Fortuny J, Belfontali V, Sadowski S, et al. Parathyroid gland angiography with indocyanine green fluorescence to predict parathyroid function after thyroid surgery. Br J Surg 2016;103:537-43. [Crossref] [PubMed]
- Vidal Fortuny J, Sadowski SM, Belfontali V, et al. Indocyanine Green Angiography in Subtotal Parathyroidectomy: Technique for the Function of the Parathyroid Remnant. J Am Coll Surg 2016;223:e43-49. [Crossref] [PubMed]
- Zaidi N, Bucak E, Yazici P, et al. The feasibility of indocyanine green fluorescence imaging for identifying and assessing the perfusion of parathyroid glands during total thyroidectomy. J Surg Oncol 2016;113:775-8. [Crossref] [PubMed]
- Lang BH, Wong CK, Hung HT, et al. Indocyanine green fluorescence angiography for quantitative evaluation of in situ parathyroid gland perfusion and function after total thyroidectomy. Surgery 2017;161:87-95. [Crossref] [PubMed]
- Vidal Fortuny J, Karenovics W, Triponez F, et al. Intra-Operative Indocyanine Green Angiography of the Parathyroid Gland. World J Surg 2016;40:2378-81. [Crossref] [PubMed]
- Zaidi N, Bucak E, Okoh A, et al. The utility of indocyanine green near infrared fluorescent imaging in the identification of parathyroid glands during surgery for primary hyperparathyroidism. J Surg Oncol 2016;113:771-4. [Crossref] [PubMed]